Around 6 p.m. each evening, the streets of Boston’s suburbs come alive with the physically fit and those aspiring to be. They are runners, bikers, walkers, and scooter riders of all different body shapes and ages who would seem to have little in common except one thing—an electronic band wrapped around their wrist. For many of these people, it’s hard to imagine life without the daily nagging from a personal health device to meet their daily prescript of 10,000 steps.
These wearable devices have become ubiquitous not just in Boston but around the world, constantly gathering personal health data such as perspiration, heartbeats, movements, acceleration, footsteps, blood pressure, sleep, and an increasing number of externally detectible bodily processes. Cisco Systems reported that, last year, 325 million wearable devices were connected worldwide, a number predicted to more than double by 2019. But their popularity has left some wondering if this sort of technology could be taken beyond the surface of the skin to places deep within our bodies—not wearable sensors but ingestible ones.
Heeding the call, materials scientists have created a new class of edible electronics, with many new devices already on the market. Proteus Digital Health, a company based in Redwood City, California, recently released an ingestible sensor for detecting whether patients have taken their medications. On their website, Proteus claims the system “measures medication treatment effectiveness and helps physicians improve clinical outcomes.” The sensor, which is roughly the size of a grain of sand, can be combined with a drug at the pharmacy that, once ingested, sends a signal to an app in the patient’s smartphone that confirms when the medication reaches the stomach. The website claims this is helpful for patients who have trouble remembering to take their medication and for doctors seeking a better way to track the efficacy of a medication. But this is just a simple sensor. Other, more complex ingestible systems are being developed that go far beyond this simple technology. They are fully functional devices that can take and communicate multiple measurements from within the body—a sort of swallowable Fitbit.
Inside Electronics
Electronics inside the body are nothing new. Implantable devices first emerged in the 1950s with the cardiac pacemaker, a device that stimulates the heart using electric pulses. Edible electronics emerged a few decades later in the late 1980s, when NASA and Johns Hopkins University developed an ingestible thermometer to gauge the core body temperature of astronauts during space walks. The device came in the form of a pill—a three-quarter-inch, silicone-coated capsule with sensors that triggered a quartz crystal to vibrate in coded frequencies, which were read by an external recorder. After 18–30 hours, the pill naturally passed from the digestive system. Edible technology took a step further in the mid 1990s with the advent of wireless endoscopes, tiny cameras packaged in an ingestible pill. This was revolutionary for gastroenterologists, who previously had no way of identifying sources of bleeding in the small intestines—a telltale indicator of conditions like Crohn’s and celiac disease. These endoscopes allowed doctors to image the entire length of the intestine, all while a patient went about his or her day.

Today, wireless endoscopes are a first-line diagnostic tool for identifying unidentified gastrointestinal (GI) bleeding. Electronics like this, which must pass all the way through the body, are great for one-time or rare use but can become problematic with regular use. The wireless endoscope is a big pill to swallow. At roughly
an inch long and half an inch in diameter, it has a 1% chance of getting lodged in the GI tract. If that happens, the patient must wait for it to pass or have it surgically removed. This is rarely a problem when a device is used as a one-time diagnostic tool but becomes a more serious issue when such devices are used therapeutically and need to be employed not once a year but every day or every other day. “Chances are one of the devices is going to get stuck,” says Christopher Bettinger, associate professor of materials science and biomedical engineering at Carnegie Mellon University in Pittsburgh, Pennsylvania (Figure 1). The question then becomes, “How do we make devices where, maybe they’re big but if they do get stuck, then it’s not even a big deal?” he continues.
Bettinger is one of several scientists rethinking how we monitor our health from the inside out. Soon after joining the faculty of Carnegie Mellon in 2010, he became interested in developing edible electronics, part of an emerging class of transient devices that are used and then absorbed inside the body. “They have allowed us to expand the application space and think about new kinds of devices that maybe aren’t doing diagnostic functions, but they’re doing therapeutic interventions,” explains Bettinger. But being able to swallow the devices has an added advantage. “Since they’re biodegradable, it’s not as risky to use them as much as every single day or every other day,” Bettinger says. And unlike transient materials that are surgically implanted in the body, edibles are given a certain degree of latitude. “Your lunch or breakfast was probably not inspected in a factory, or sterilized, or hermetically sealed,” like an implantable device would be, he continues. As long as you can ingest the materials that compose the device, the time line for U.S. Food and Drug Administration approval is greatly reduced, from ten or more years for testing to just a few, Bettinger states, citing Proteus’s sensor pill, which was approved after just four years. Faster approvals leave more time for innovation, which breeds room for creativity, Bettinger says.
Powering Up
Making an entirely ingestible device is a challenge. Bettinger started off by reimagining the key functional components of the wireless endoscope—the packaging and the battery. He began with the battery. Batteries function by exchanging ions between a cathode and an anode, essentially the positive and negative ends. The positive end is made up of a material containing an excess of ions, and the negative end contains a deficiency. Once the two ends are connected using a conductive material like copper, they exchange electrons, and those traveling ions power a circuit along the way. The key is to identify an easily ionizable material like lithium. Melanin, a pigment found in our hair, skin, and eyes, was the perfect candidate for the job. Much like lithium, melanin appears in the body naturally and isn’t toxic, so it can be absorbed. Bettinger designed a battery that essentially replaces lithium with melanin and substitued biodegradable materials, like saltwater, for other components. And it worked.
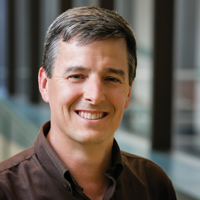
The next step was to figure out how to package the device. Bettinger needed a packaging material that was both biodegradable and flexible. In 2012, John Rogers, a professor of materials science and engineering at Northwestern University in Chicago, Illinois (Figure 2), discovered that silicone, a material used to encase electronics, is ingestible and dissolves slowly in saliva and other body fluids. Even better, Rogers was able to define silicone’s rate of decomposition within the body. He found that by adjusting the thickness of the silicone, he could adjust the rate of decomposition. That meant he could program materials made of silicone to be active for set periods of time within the body, like a dissolving suture that is necessary only until a wound heals. But in this case, he says, “It’s a full electronic device that has a resorbable characteristic.”
Rogers recently used silicone to develop a device for monitoring brain function in patients with severe traumatic brain injuries. The device is inserted into the intracranial space inside the skull, where it applies pressure and monitors temperature and electrical signaling during the highest risk period after the injury. Once it’s no longer needed, it dissolves and is absorbed in the body, negating the need for surgical removal. Other devices he’s created act as programmable drug-release vehicles or deliver electrical shocks to relieve pain. Silicone opens many doors because “you can leverage a lot of knowledge from the silicone consumer electronics world,” Rogers says.

This was precisely the kind of material Bettinger was looking for to encase his battery. But Rogers has only used silicone for devices that are implanted in the body and not swallowed. Bettinger’s initial tests suggest the battery should work well though. The first version he developed in 2013 remained operational for five hours, but subsequent versions lasted three times that long (Figure 3: Photo courtesy of Stephanie Strasburg, Pittsburgh Tribune). So far, he has not tested the battery in the human body, but he says he hopes to within the next few years. After the battery has been tested and approved, it is Bettinger’s hope that it could be used to power any device a clinician might want to employ, including a camera.
Other Edibles
Silicone and melanin aren’t the only materials that can be used in edible devices. Hanqing Jiang, a professor of mechanical and aerospace engineering at Arizona State University in Tempe (Figure 4), and his collaborators recently published a tool kit of food-based electronic materials for developing new edible devices. They tested foods for electrical conductivity and then separated them into a convenient food pyramid chart, grouping each food according to its idealized role in a circuit. Dried vegetables mixed with flour and oil conducted electricity poorly, they found, which made them useful for electrical insulation. Foods with high water and salt content, like fish and salted butter, tended to be more conductive and better for circuit wiring.
Interestingly, the most conductive materials tended to be processed foods, which contained higher levels of conductive, edible metals. Burned, or carbonized, cotton candy turned out to be particularly conductive. “Once you carbonize something like cotton candy or a marshmallow, it becomes pure carbon, and carbon is conductive,” says Jiang. By adding flour and water to the burned candy, he and his colleagues created a dough that they pressed into a noodle, sprinkled with edible gold flakes, and then used as a wire.
They also constructed circuit boards out of sugar paste and capacitors out of thin gelatin sheets. As Jiang explains, “We found that broccoli is one of the most cellulose-rich vegetables we eat,” a key attribute for making piezoelectric materials that produce electricity when subjected to mechanical stress. Some microphones use pliable materials like this, which flex when exposed to sound waves and generate voltage that can be channeled through a circuit and then played through a speaker. The team tested this concept by building a microphone composed of a thin film made of dried and finely ground broccoli powder mixed with gelatin. Interestingly, they used it to record the bowel sounds of a 70-year-old man, which were subsequently blasted through a loudspeaker. Their justification, they argue, was that low-frequency sound can be used to identify pathological conditions in the gut. Jiang plans to build more operational devices over the next two years. “If we can make a device that effectively works in the GI tract, “he says” then we will have created something that’s transformative.”
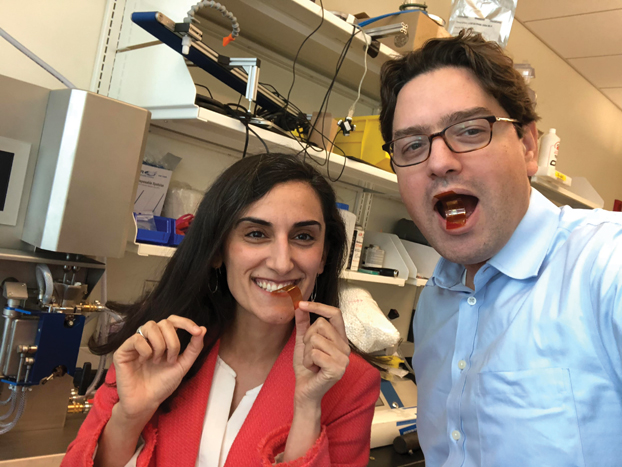
Progress is also being made in the nonbiodegradable edible electronics space. Giovanni Traverso, a gastroenterologist and biomedical engineer at Brigham and Women’s Hospital in Boston, Massachusetts, teamed up with Canan Dagdeviren, a materials scientist at the MIT Media Lab in Cambridge, Massachusetts (Figure 5: Photo courtesy of Farhad Javid), to develop an ingestible device for measuring movement in the stomach. Their device is made of piezoelectric material, which generates a charge by simply moving through the gut. It is rolled up into a capsule that is swallowed and dissolves once it reaches the stomach. Free in the gut, it unrolls and electrostatically adheres to the stomach lining, where it naturally expands and contracts as food is ingested and digested. This motility does double duty—it both provides a reading in the device and powers the device just by moving. It also enables the device to power itself in the harsh environment of the stomach without the use of chemicals and without the risk of electrical and mechanical degradation, says Dagdeviren.
This is a game-changer for gaining insight into gastroparesis, a GI issue that makes patients unable to effectively propel ingested materials forward through their gut—a common symptom of diabetes. This inability leads to an accumulation of food and liquid in the stomach, which causes nausea and vomiting. Traverso and Dagdeviren tested the device in pigs, where it remained active for two days, says Traverso, “and that was really surprising to us, but also reassuring, as it really potentially supports the ability to use these systems for long periods of time.”
Expanding the Application
As more and more components for edible devices are proven to work, scientists are beginning to think beyond the initial testing phases to potential applications. Traverso and Dagdeviren believe their technology might benefit both patients and doctors who wish to monitor the frequency and quantity of food ingested into a patient’s stomach—a useful tool for patients struggling with obesity. “I think the most exciting uses are in the diagnostic and medical base,” says Bettinger, who plans to soon test sensors and combinations of sensors powered by his battery within the body. He hopes to eventually create a device that measures metabolites, substances produced after digestion, in real time to determine how individual foods impact digestion. He also thinks the technology could be used to determine how the bacterial ecosystem in the gut is linked to diseases like celiac and inflammatory bowel disease.
There are countless applications, Bettinger notes, and “one’s imagination is sort of the limit.” Rogers agrees, citing the many uses of silicone in the consumer electronics world. These types of devices “are not a sci-fi concept anymore,” he says; from the standpoint of technological capabilities, the future looks very bright.”
References
- Cisco. (2017, Mar.). Cisco visual networking index: Global mobile data traffic forecast update, 2016–2021. Cisco. San Jose, CA. [Online]. Available: www.cisco.com/c/en/us/solutions/collateral/service-provider/visual-networking-index-vni/mobile-white-paper-c11-520862.html
- Proteus Digital Health. (2017). Proteus discover. Proteus Digital Health. Redwood City, CA. [Online]. Available: www.proteus.com/discover/
- W. Greatbatch and C. F. Holmes. “History of implantable devices,” IEEE Eng. Med. Biol. Mag., vol. 10, no. 3, pp. 38–41, Sept. 1991.
- B. Dunbar. (2006). “Thermometer pill” helps athletes, firefighters and others beat the heat. NASA. Washington, D.C. [Online]. Available: www.nasa.gov/topics/nasalife/thermometer_pill.html
- T. Nakamura and A. Terano, “Capsule endoscopy: Past, present, and future,” J. Gastroenterology, vol. 43, no. 2, pp. 93–99, 2008.
- G. D. Bakhshi, M. B. Tayade, K. V. Jadhav, D. D. Choure, N. L. Mane, and S. R. Patil, “Retention of an endoscopic capsule,” J. Minim. Access Surg., vo. 10, no. 3, pp. 163–165, 2014.
- B. Edwards. (2012, Aug.). After four year wait, Proteus earns FDA approval for ingestible pill sensor. IMedicalApps. [Online]. Available: www.imedicalapps.com/2012/08/proteus-digital-health-fda-approval-ingestible-pill-sensor/
- W. Xu, H. Yang, W. Zeng, T. Houghton, X. Wang, R. Murthy, H. Kim, Y. Lin, M. Mignolet, H. Duan, H. Yu, M. Slepian, and H. Jiang. (2017, Sept.). Food-based edible and nutritive electronics. Adv. Mater. Technol. [Online]. Available: onlinelibrary.wiley.com/doi/10.1002/admt.201700181/full