Since stem cells give rise to all the different cell types that make up our bodies, they have the potential to repair or replace cells that are missing or dysfunctional in a wide range of diseases and injuries. In recent years, an explosion of clinical trials involving stem cell therapies has inspired hope that such regenerative strategies may soon cure some of our most vexing diseases. Before that hope is realized, we will need a greater understanding of the fundamentals of stem cell biology as well as the specifics of different disease processes. Although the challenges seem daunting, stem cell research is rapidly advancing and ushering in a new era of regenerative medicine.
What are stem cells?
There are different types of stem cells that come from different places in the body or are formed at different times in our lives, but they all have two key characteristics: the ability to make copies of themselves, or self-renew, and the ability to differentiate, or develop into more specialized cells.
One type of stem cell is the embryonic stem cell, which exists only at the earliest stages of development. Embryonic stem cells come from embryos that are three to five days old. At this stage, they are called blastocysts and consist of a mostly hollow ball of about 150 cells. Human embryonic stem cells are derived primarily from blastocysts that were created by in vitro fertilization for assisted reproduction but were no longer needed. Embryonic stem cells are pluripotent, meaning they can generate all of the body’s cell types.
Other types of stem cells are multipotent, meaning they can generate just a few different cell types, generally in a particular tissue or organ. There are various kinds of multipotent tissue-specific stem cells, also sometimes called adult stem cells, that appear during fetal development and remain in our bodies throughout life. Tissue-specific stem cells have been found in some organs that continually replenish themselves, such as the bone marrow, skin, and gut, but have also been found in other, less regenerative organs, such as the brain. These types of stem cells can be difficult to identify and isolate in the human body and are more challenging to grow in culture than embryonic stem cells.
The turning point in stem cell research came in 2006, when Takahashi and Yamanaka [1] discovered that it was possible to reprogram adult cells to the pluripotent state. The scientists used viruses to insert extra genes, mainly expressed in embryonic stem cells, into adult skin cells. They named this new form of stem cell induced pluripotent stem (iPS) cells.
Stem cells in research
The accessibility of iPS cells, compared to embryonic stem cells and adult stem cells, opened up the field of stem cell research. Lab-generated iPS cells now serve as critical tools for researchers seeking to learn more about normal human development as well as disease onset and progression.

“Not all events that happen in mouse development occur in humans, so stem cells offer a way to study these developmental processes in human cells,” says Jennifer Davis, associate director of the Institute for Stem Cell and Regenerative Medicine at the University of Washington. “Understanding how the fate of a stem cell is decided, and learning how to control those fate determinations, tells us a lot about how human beings develop.”
One major advantage of iPS cells is that they are a good way to make pluripotent stem cell lines that are specific to a disease or even to an individual patient. Disease-specific stem cells are powerful tools for learning more about the cause of a particular disease as well as testing drugs to treat that disease.
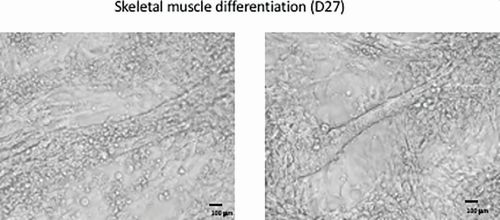
In the lab of Gabsang Lee (Figure 1), a neuroscientist at Johns Hopkins School of Medicine, patient-generated stem cells are used to study the mechanisms of peripheral nerve diseases and muscular dystrophies and to test therapeutic compounds (Figure 2). “If you get the cells from a patient, like a patient with amyotrophic lateral sclerosis (ALS), you can generate motor neurons from those cells and test compounds in that patient’s motor neurons,” says Lee. “In this way, you can learn about whether a drug may work in a particular patient.”
Stem cell therapies
The ability to generate patient-specific stem cells is also attractive for cell therapy [2]. In a stem cell transplant, stem cells are first guided into becoming a specific cell type that is required to repair damaged or destroyed tissues. Then those cells are transplanted into the patient, where they take the place of the tissue damaged by disease or injury. This type of treatment could potentially be used to replace any tissue or organ in the body, and when the cells are derived from the patient him- or herself, the risk of immune rejection is minimized.
Currently, very few stem cell treatments have been proven safe and effective. The most commonly used stem cell treatment is hematopoietic (or blood) stem cell transplantation, for example, bone marrow transplantation, to treat certain blood and immune system disorders, such as leukemia.
Most other stem cell treatments, while promising, are still in the experimental stages. In the past few years, there has been an exponential advancement in clinical trials revolving around stem cell-based therapies. Some of these trials have already generated impressive results. For instance, patient-derived iPS cells were induced to differentiate into pigment epithelial cells of the retina, which, when transplanted into patients with macular degeneration, improved eyesight. Other clinical trials are targeting diseases and injuries including cancer, Parkinson’s disease, stroke, osteoarthritis, and traumatic brain injury [3].
Jeffrey Millman and his team at Washington University St. Louis work on generating insulin-producing beta cells of the pancreas to treat diabetes (Figure 3). It wasn’t until 2014 that Millman and his colleagues figured out how to coax stem cells into becoming beta cells. Now, their work is focused on improving that complex process.
“There are still issues with the beta cells we make,” he says. “Even though they make and secrete insulin in response to sugar, they are immature in the way they do it. Recently, we have been able to make more mature beta cells, with a much higher yield, and they cured diabetes better and faster when transplanted into diabetic mice.”
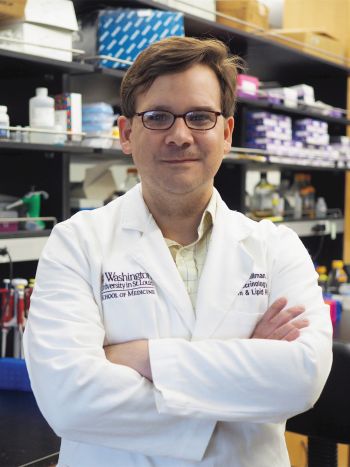
Challenges remain
Despite the successes and advancements of recent years, there are several major challenges that must be addressed before stem cell therapies become available to treat a wide range of diseases.
The first issue is safety. Transplanted stem cells, like any transplanted organ, can be recognized by the immune system as foreign and rejected. Using a patient’s own iPS cells may lessen this risk, but even these cells may not entirely escape the notice of the immune system. In addition, there are concerns that iPS cells could induce tumor formation due to the accidental expression of oncogenes when the cells are being reprogrammed [3].
Lee says that recent developments, including CRISPR/Cas9 gene editing technology, have largely allayed his fears about stem cell safety. “We know what kinds of genes are causing immune rejection and inducing tumors,” he says “By using the CRISPR/Cas9 gene editing system, we can selectively delete those problematic genes and create a cell that is safer and tolerated by the immune system,” he says.
The second issue is one of sourcing and scaling. While iPS cells can be grown indefinitely in the lab, the processes by which they differentiate are complex and must be tightly controlled. The efficiency of making specific cell types from iPS cells must be improved before stem cells become a reliable and routine therapy.
The scale of the procedure is another challenge. “How do you take a scalable process that works on a petri dish of one to ten million cells and make it work for a billion cells for one patient, or a trillion cells, for one thousand patients?” says Millman. “This is a challenge in bringing these treatments to more people.”
A third issue is getting transplanted stem cells to their intended place and encouraging them to fully integrate with the body’s other cells. Cells must be delivered to the right part of the body and, once there, must be able to fully replace lost or malfunctioning cells.
Davis says this is a challenge in using stem cells for cardiac regeneration, though she and others are working on solutions. “When new heart muscle cells are transplanted into the host, they have to integrate with an organ that is already developed,” she says. “And in the heart, they not only have to integrate with the vasculature that is already there, but they also have to integrate electrically.”
Promise of stem cells realized
It’s now been 15 years since the ability to generate iPS cells was hailed as a game changer for regenerative medicine. Some may wonder, when will more stem cell therapies become available?
“It sounds like a lot of time, but in the scale of medicine and science, that is incredibly rapid,” says Millman. “The worst thing we could do is rush into putting cells into people and hurt somebody. It would be tragic for the individual hurt, but it would also be a disservice to the millions of patients who could benefit from cell therapy by setting the field back for a time.”
Even with the challenges remaining, stem cell therapy is becoming a more tangible reality by the day. Lee points to the many ongoing clinical trials applying these approaches to different diseases. “We’re almost there, with some diseases,” he says. “If trials go well, we might be looking at new therapies in a few years.”
Millman, too, is optimistic about the potential of stem cell-based therapies to help a great many people in the future. “I got into stem cells because of how much promise was there,” he says. “I am enthusiastic and excited about what this will mean for people one day. But it’s not here yet.”
References
- K. Takahashi and S. Yamanaka, “Induction of pluripotent stem cells from mouse embryonic and adult fibroblast cultures by defined factors,’’ Cell, vol. 126, no. 4, pp. 663–676, 2006, doi: 10.1016/j.cell.2006.07.024.
- W. Zakrzewski, M. Dobrzyński, M. Szymonowicz, and Z. Rybak, “Stem cells: Past, present, and future,’’ Stem Cell Res. Therapy, vol. 10, no. 68, pp. 1–22, 2019, doi: 10.1186/s13287-019-1165-5.
- R. M. Aly, “Current state of stem cell-based therapies: An overview,’’ Stem Cell Invest., vol. 7, no. 8, 2020, doi: 10.21037/sci-2020-001.