When soft robotics first emerged, it was defined (as breakthroughs often are) by what it was that its traditional counterparts were not, i.e., soft. A decade in, the nomenclature remains apt. The pliant materials used in soft robotics are often both a defining trait and a source of advantage. Soft robots continue to make headlines with their ability to squeeze, octopus-like, through narrow crevices; change shape; and survive impacts that would crush a traditional rigid robot. They distinguish themselves from their hard counterparts with their damage resilience, complex movements, and suitability for use with the human body.
But as with many bipolar categories like soft and hard, it’s in the in-between gradient areas that interesting hybrids and category-bucking solutions are found. The dating of soft robotics varies, but there’s some consensus that the field is about ten years old. Many point to the U.S. Defense Advanced Research Projects Agency (DARPA) chembots and programmable matter projects as early examples. As this nascent field develops, its robots are evolving. It used to be that the defining objective was for all components to be soft (with the idea of achieving maximum morphing capability). Today, that goal has loosened, with some researchers focusing on the interplay between hard and soft materials. Some soft robots now integrate skeletal components, while some use variable-stiffness materials; yet others seek to move from hard to soft more seamlessly through the design of actuators and sensors.
As soft robotic solutions proliferate, their sources of inspiration multiply as well. Worms and cephalopods have reigned as the prototypical models and continue to prompt innovations, such as the headline-grabbing 2016 Octobot from Harvard University and the microrobot-caterpillar that mimics natural caterpillar locomotion from the University of Warsaw, Poland. Soft robots are also now taking bioinspiration from other, less totally soft organisms (including humans), and one recent example departs from nature altogether, drawing inspiration instead from origami. That particular robot, a joint effort by the Massachusetts Institute of Technology (MIT) and Harvard, astonished the world by lifting 1,000 times its own weight without requiring a control system.
The Goldilocks Grasp of the Soft Robot
“It would be great if our robots behaved more like us,” says Rob Shepherd. Shepherd runs the Organic Robotics Lab at Cornell University, which describes itself as “using synthetic adaptation of natural physiology to improve machine function and autonomy.” When Shepherd says robots should be more like humans, he doesn’t mean that we are perfect; he means that our bodies work well in the environments we inhabit and create. We can, for example, squeeze an avocado to test for ripeness without crushing it if it turns out to be overripe. Could a robot do this? A soft robot could, yes. This refinement of touch is feasible due, in part, to the materials they’re constructed from, and their pliancy, some argue, makes them more like humans than hard robots.
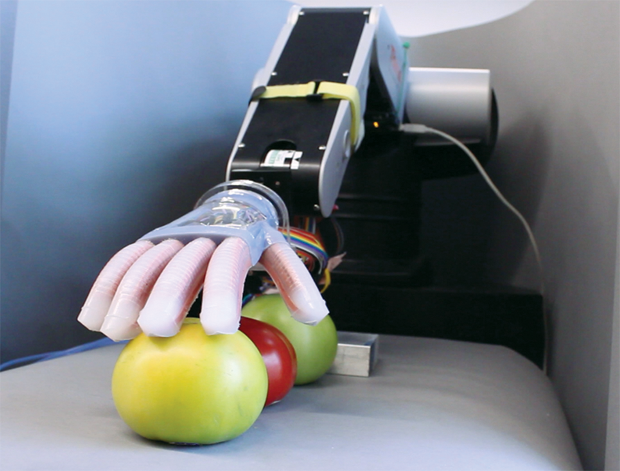
Soft robotics hardware can also tolerate environmental imperfections better than a rigid robot might. Imagine a robot manipulator that has been virtually trained by a simulator, such as tech company Nvidia’s Isaac, to pick up an egg. Because the real world has more variables than a computer environment, a very precise manipulator may pick up a virtual egg without a hitch but then go on to break or drop an actual egg in the real world. With compliancy built into that manipulator, suggests Shepherd, “it can be off a little bit and still pick up that egg. It won’t break it. It won’t drop it.”
Shepherd has been developing optoelectronic elastomeric strength sensors for multiple applications, including equipping a prosthetic hand with a more refined sense of touch. A prototype of this hand has demonstrated its ability to pick out the ripest tomato from a lineup of three by sensing which is the softest (Figure 1) [2]. The hand’s optical sensors operate by what Shepherd describes as a rubber lightguide (Figures 2 and 3). “By measuring the light output of a rubber optical waveguide,” he explains, “the degree to which it is stretched can be measured. The more the lightguide is stretched, or otherwise deformed, the dimmer it will be. The chemistry and shape of the lightguide can be tuned for the application.” In the example of the robotic hand detecting differences in ripeness among the three tomatoes, the very soft and thin configurations enabled by the lightguide deform to detect those differences in stiffness without damaging the fruit’s skin.

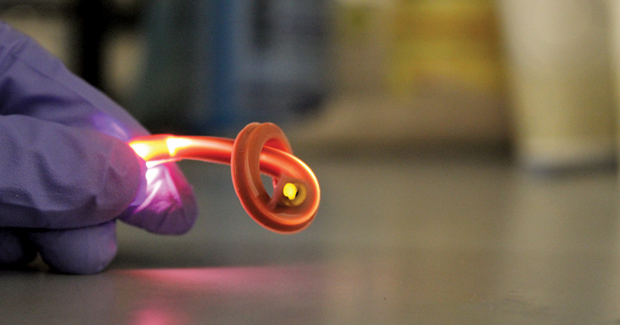
Robots That Do Things with Us, Not Just for Us
In a world where robots are starting to do things with us and not just for us, soft robotics is a good fit for prosthetics and wearable technology, literally because it fits bodies better. The parts are often very lightweight and have less abrasive interfaces to interact with than a traditional motorized robotic system. “A motor is incredibly brutal in its operations. It will only go in one way, and it will only do one thing,” says Jonathan Rossiter, professor of robotics at the University of Bristol, United Kingdom. Soft robotics materials, on the other hand, can move in several different ways. “They can bend and they can twist and they can curl up and they can stretch lengthwise like a muscle,” Rossiter continues. All are useful movements when interacting with a human body.
When he was a master’s degree student at MIT with Hugh Herr, Conor Walsh worked on a rigid exoskeleton and wore it many times to test and evaluate its performance. Similar commercial systems have enabled patients with paralysis from spinal cord injuries to take steps again and even walk with the assistance of the exoskeleton. This experience got Walsh thinking about other patients with less severe injuries who retain some ability to walk, e.g., stroke patients. For these people who can still walk but do so very slowly or inefficiently, the traditional rigid suit seemed like overkill. “So,” recalls Walsh, “we came up with the idea that if we could give small boosts at the right time, maybe just to the ankle or to the hip, or maybe both, and with a very lightweight and nonrestrictive system,” then the quality of life could be improved for those patients who were able to walk but with great difficulty. That was about five years ago. Since then, Walsh’s soft exosuit has made headlines for its inroads into wearable technology (Figures 4 and 5) [3].
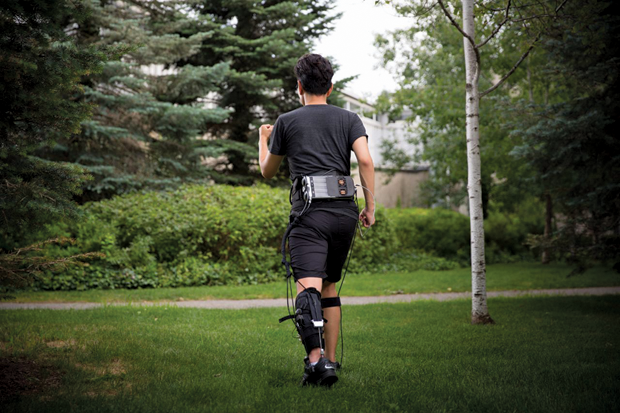

Today, Walsh is a professor of engineering and applied sciences at Harvard University and a core faculty member of its Wyss Institute. He made Popular Science’s “Top Ten Most Innovative Minds in Science and Engineering” in 2016.
The design for the suit, essentially a wearable robot, was based on walking being a dynamic process, characterized by an “inverted pendulum-type motion,” as Walsh describes it. Like gently pushing a person on a swing, the soft exosuit delivers “a little tap or a boost at the right time to maintain the system’s movement,” he says. His team’s focus was on the ankle joint, where one might find most walkers’ deficits (Figures 6 and 7).
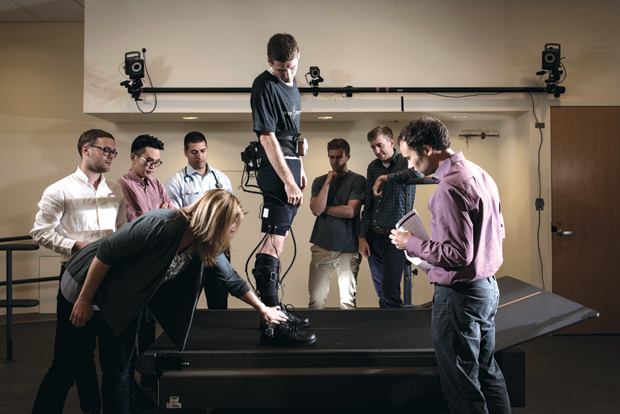
Walsh wanted a close synergy and interaction between the suit and its wearer rather than a suit that would dominate the person’s system with force, which is what rigid exoskeletons do. His objective presented controls challenges, e.g., how to use sensors in the suit to apply the right force at the right time. “The way we do that,” he explains, “is we monitor how a person is moving, and then we have an understanding of what the normal muscles and tendons are doing during walking.” That information is used to ensure the cables pull at the right time to mimic what the muscles are doing. To maximize the suit’s benefits, some of the control parameters are individualized to the person wearing it. The suit detects key walking events, such as when the wearer’s heel hits the ground, when the heel lifts up off the ground, and when the walker is pushing forward. As Walsh explains, “We have algorithms that learn how a person is walking and automatically fine-tune some of those [walking events] for each individual person.”

Walsh’s team hopes to increase the suit-wearer’s walking speed from an average of 0.3 to 0.6–0.8 m/s (a healthy gait is typically 1.2 m/s). Additionally, compared to healthy individuals, stroke victims can expend as much as 70% more net energy on walking [3]. “With data, we’ve shown that we can reduce energetics [of the person wearing the suit], and also improve their symmetry, or the pattern of their gait,” Walsh says. Although designed to improve walking ability, the suits have the potential to do more. A greater ease in walking tends to have a positive, cascading effect on this population’s quality of life, allowing them the benefits of exercise and overall greater integration into the daily life of their communities. The suit could also stave off the atrophy that comes with impaired walking.
Like Shepherd’s prosthetic hand and other soft robots, Walsh’s suit brings a lighter touch than its rigid antecedents in both construction and design. Made of ultralightweight, flexible materials, it applies the right amount of force (that tap or boost) at the right moment, to aid with the specific walking challenges of stroke victims. Future iterations, says Walsh, may be beneficial to those who suffer from multiple sclerosis and Parkinson’s disease as well. In the world of human–robot interaction via wearables, less—less bulk, weight, and force—provides more.
Soft Robots Inside the Body
Soft robotic devices are being envisioned for use in internal medicine as well. They could bring more degrees of freedom and greater mechanical flexibility to surgical tools. They could be edible robots for medicine delivery or data gathering. They might also be implantable devices to assist with organ function. One advantage they have is that pliant materials can be more readily engineered to have properties in common with biological tissue.
“Squishy, Adorable, and Revolutionary,” announced a New York Times headline in August 2016, introducing readers to Octobot, the world’s first untethered, fully autonomous soft robot. Made from silicone gel using three-dimensional printing, it looks, as its name implies, like a rubbery little octopus and is a far cry from the early DARPA chembot, which elicited reactions of “creepy” from more than a few people. Pint-sized and translucent, Octobot’s blue and red wiring shows through its skin-like veins. Cordless and battery-free, with no rigid power source in its belly, the Octobot is powered by chemical reactions that produce a gas enabling it to first raise four of its limbs, then the other four limbs, and so on, in a kind of wiggle that propels it along. It’s controlled by a microfluidic logic circuit that acts like a circuit board, directing the flow of fuel. Octobot signaled a host of new applications for soft robots in health care, including future incarnations in which, constructed from biodegradable and biocompatible materials, it might be swallowed in capsule form to perform a much less invasive endoscopy, for example.
Heart function is also receiving an assist from soft robotics. Shepherd’s lab is developing a method for molding actuators three-dimensionally into complex geometries that could be used to noninvasively compress an ailing heart. Using a computed tomography, or CT, scan of a person’s heart, Shepherd’s team has molded foam around a printout of the scan. The foam has interconnected pores through which a fluid can pass to pressurize the foam rubber, altering its stiffness and shape. The foam can be patterned so that it pressurizes only in areas where there aren’t major coronary arteries, to assist a weak heart with pumping and blood flow.
Also with the aim of supporting cardiovascular functions in a weakened heart, Walsh’s lab at Harvard has collaborated with Boston Children’s Hospital to make a robotic cardiac sleeve [4]. The sleeve encases the heart externally, and its actuators, or pneumatic artificial muscles, are programed to pump with the patient’s weak heart in motions that mimic those of a healthy heart, twisting and compressing with each pump. The sleeve improves upon existing ventricular-assist devices by not coming into direct contact with the heart’s blood flow, thereby reducing the risk of clotting and stroke. Another potential benefit of the robotic sleeve, although not yet fully proven, is that its compression acts to massage some of the border-zone at-risk heart muscle, potentially salvaging it. This at-risk muscle, still viable after a cardiac failure, often suffers in an adverse cascade of events because it is working harder and overcompensating for the permanently damaged muscle. As presented in its January 2017 proof of concept, the sleeve could act as a bridge to transplant but will require more development before it can be implanted for longer-term support.
One of the lead researchers at work on that longer-term implantable version of the sleeve is Ellen Roche, professor at MIT’s Institute for Medical Engineering and Science. With a background in bioengineering medical devices, Roche began her Ph.D. degree work at Harvard just as soft robotics was starting to take off. She couldn’t stop thinking about how soft robotics was a natural fit for medical devices. “You can make things change shape and grow over time, which is really interesting for implantables because that’s obviously not possible with something rigid that you implant,” she notes. With soft robotics, “there’s a whole new way to think about making things adaptable, atraumatic, and very biomimetic.”
Drawing on Nature in Soft Robotics Design
Biomimicry and bioinspiration underlie many of the most innovative soft robots. When the mini caterpillar robot debuted in 2016, one of its creators, Piotr Wasylczyk, said in a press release, “We are only beginning to learn from nature and shift our design approaches toward these that emerged in natural evolution.” Ask roboticists about the degree to which biomimicry factors into their projects, and you’ll hear a range of responses. This could depend on where their focus lies—whether they’re working to solve a motion, material properties, or morphology challenge. In designing the movement of the cardiac sleeve, for instance, Roche took a selective approach, focusing on biomimicry when it came to the motion of the robotics. She wanted to engineer, as closely as possible, the specific movements of a healthy human heart. “But in general, for design and materials,” she says, “I don’t think it has to be exactly mimicking the organs that are there already. You can simplify a lot. What I try to do is to define the motion and then mimic it using the minimal amount of actuators that I can.” The contractions of real muscle tend to be so tiny that “it’s almost impossible to recreate them for devices,” says Roche. With devices, simpler is better, so she asks herself, “What’s the best and simplest configuration to achieve the same end result?”
Named in Forbes’s 2015 list of “30 Under 30” for her research in soft materials, Rebecca Kramer-Bottiglio, who leads the Faboratory at Yale University, agrees that creating biomimetic systems is incredibly difficult. Trying to replicate “the way that our muscles work and the way that our sensory systems are integrated within our bodies” is an “open-ended challenge,” she says. In 2017, Kramer-Bottiglio was awarded a young investigator research program grant from the Office of Naval Research for her design of a morphing robotic limb. The limb challenges the soft/rigid robotics binary while showing that bioinspiration can come from multiple sources to produce a hybrid form.
Kramer-Bottiglio found inspiration in the morphologies of the turtle and the tortoise. By employing variable-stiffness materials and a reconfigurable actuation system, her morphing limb is able to shape-shift from a flipper optimized for moving in water (like a sea turtle) to a leg optimized for moving on land (like a tortoise). Variable-stiffness fluidic bending actuators are distributed along the limb and reconfigure to move from a thin, flat state (flipper) to an inflated, bent state (leg) (Figure 8).

Kramer-Bottiglio’s robotic, amphibious turtle, referred to more formally as a biomimetic unmanned, untethered vehicle, is “ultimately not going to have a flipper that is anatomically exactly like a turtle flipper or a leg that’s exactly like a tortoise leg,” explains Kramer-Bottiglio. “It’s going to have a hybrid design that can morph between these two general limb configurations to achieve some kind of efficient locomotion in both land and sea environments, while being able to accommodate that morphing capability.” This morphing capability goes beyond what nature has supplied in either creature, showing how innovations often emerge from bioinspiration rather than exact biomimicry. “Animals are an incredible source of inspiration,” says Kramer-Bottiglio in talking about her creative process. “Often, it’s more helpful to look at their high-level morphologies and behavioral policies, rather than the anatomical details,” she says, of designing robotic systems and the ways they move.

How might the soft robotics behind an amphibious unmanned, untethered vehicle have a future in healthcare solutions? The innovation to watch for in this case is in the variable-stiffness materials Kramer-Bottiglio and others are working with, and the capacity for a robot to manifest in one state, soften, and then morph into another state. (See one example in Figure 9.) The implications for wearables are promising. This technology could transform the conventional cast as we know it, offering the option of “on-demand” stiffness in a limb brace, for example. Imagine being able to wrap a leg with variable-stiffness materials, says Kramer-Bottiglio, “which could be removed, repositioned, and tuned for optimal healing and comfort.” Variable-stiffness materials could also be incorporated into bionic and prosthetic limbs, she says, introducing the possibility “to actively tune stiffness and morphology, ever so slightly, to enhance efficiency during different gaits or in different environments.”
Blurring the Line Between Hard and Soft
Meanwhile, soft roboticists are also incorporating skeletal elements into otherwise soft robots, suggesting that the dividing line between soft and rigid, while probably never going away, may increasingly blur as materials science develops more materials that can change state—and, by extension, perform—multifunctionally. Shepherd says that he feels fine about incorporating skeletal elements alongside soft elements “as long as it’s in a distributed way.” Humans model this in our own shape: a hard endoskeleton with a soft exterior. “There are benefits to having the range of motion of the musculature,” points out Shepherd. “There are also benefits to constraining those degrees of freedom with skeleton,” he adds.
The profile of soft robotics research teams is also changing. The three traditional robotics disciplines (mechanical, electrical, and computer science) are collaborating with new partners, such as apparel and industrial designers. “We’re all exploring this rich space right now,” says Shepherd. “Trying to come up with the right kind of actuators, the right kind of sensors, the right kind of skeletal design. … It’s a materials challenge. It’s a controls challenge. It’s a machine design challenge.” Further subcategories of challenges are drawing researchers’ attention, such as self-healing and damage resilience, the “contradiction” between endurance and agility, energy efficiencies, and multifunctional materials. It’s an incomplete list, but it indicates how the field, barely a decade old, continues to see vibrant growth. Soft robots may never entirely oust their rigid cousins, but they will certainly spread, their shape-shifting acting as a kind of hat-tip to evolution itself.
References
- B. T. Quinlivan, S. Lee, P. Malcolm, D. M. Rossi, M. Grimmer, C. Siviy, N. Karavas, D. Wagner, A. Asbeck, I. Galiana, and C. J. Walsh, “Assistance magnitude versus metabolic cost reductions for a tethered multiarticular soft exosuit,” Sci. Robot., vol. 2, p. 1, Jan. 2017.
- Science Magazine. (2016, Dec. 12) A spongy robot hand with a sense of touch. YouTube. [Online].
- Harvard University. (2017, July 26). Soft exosuit for post-stroke gait retraining. YouTube. [Online].
- SciNews. (2017, Jan. 18). Soft robot helps the heart. YouTube. [Online].