It seems as if many of us are getting used to the idea of wearing sensors, whether they are counting the number of steps we take each day with an iPhone-connected pedometer or keeping track of heart rate while we are doing an exercise routine or out riding a bicycle. That’s probably good preparation, because a new generation of wearable sensors and smart textiles are coming to the fore to provide extended biomedical monitoring of heart, brain, muscle, and other physiological activity.
These wearable technologies are taking many forms and are in varying stages of development. Some may never make it to a commercial product, but they provide an idea of the range of devices that are under way in the burgeoning field of wearable sensors and smart textiles with biomedical applications.
Keep it Dry
Behind many of these new technologies are dry sensors. While the standard gel-based electrodes used in medical facilities today work well for short clinical sessions, dry sensors offer long-term, continuous, and ambulatory monitoring capabilities.
“The conductive gel that provides the contact between the standard electrode and skin irritates over time, and even more so, the gel itself dries, so the contact quality degrades very quickly,” says Gert Cauwenberghs, professor of bioengineering at the University of California San Diego (UCSD) Jacobs School of Engineering and codirector of the UCSD Institute for Neural Computation. What makes dry sensors versatile is that they eliminate the dependency on gel, offering a practical solution for making electrophysiological sensors wearable for the long term.
There are two major types of sensor electrodes that eliminate the gel and rely on high-impedance coupling to the skin: dry-contact electrodes that sit against the skin and noncontact electrodes that perch near but not on the skin [1]. Strong capacitive coupling over short distances complement the weak conductance through dry skin, clothing, or hair, allowing both types of electrodes to pick up the tiny voltages generated from the body [2]. “Typically, for a few millimeters of spacing, as you would have through thin clothing or normal hair, the signal can be quite reliable,” Cauwenberghs explains.
Research labs around the world are exploring ways to perfect dry-sensor systems. Much of that work today centers on using the systems to monitor the brain and the heart. A few systems are already on the market, including the HD-72 Dry Wireless Electroencephalogram (EEG) Headset System offered by San Diego–based Cogionics Inc., a spinoff company that grew out of technology developed at UCSD, and a set of headsets by Wearable Sensing of San Diego, which is a spinoff of technology originally generated by Quantum Applied Science and Research (QUASAR) Inc.
Catching the (Brain) Wave
Cogionics’ EEG headset (see above) is a series of connected bands outfitted with a built-in, wireless EEG amplifier and dozens of dry-contact sensors, along with a small battery pack that sits at the neck. This includes up to 72 sensor positions plus a three-axis accelerometer. “These are biopotential electrodes that can operate through hair to collect signals. That is critical for nonintrusive, brain–computer interfaces,” says Cauwenberghs, who is also the founder and chair of the scientific advisory board for Cogionics.
The 72-Channel Dry EEG Headset System includes a highly optimized amplifier, which combines high-input impedance, shielding, and a high-resolution analog-to-digital converter. “The bottom line is that we have such an amplifier that detects those small signals from the body, even though coupling impedance can be rather weak. In addition, the amplifier is also low noise and low power, which ensures that we can collect the signal with minimum battery usage,” Cauwenberghs says. The result is a compact and low-weight, mobile wearable system (below).
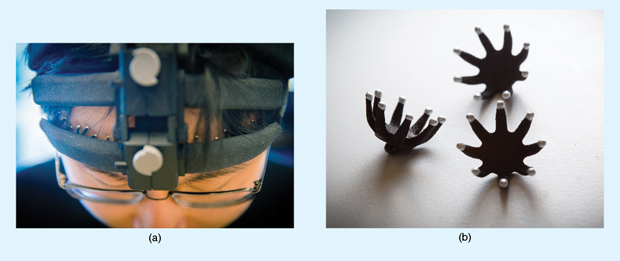
Incorporated in 2010, Cogionics took the technology from the lab to the marketplace, and that included refining the sensor prototypes. “The dry-contact ‘flex sensors’ we build today (below) are quite different from the prototypes. We have definitely gained a lot of know-how,” says Yu Mike Chi, who was a doctoral student under Cauwenberghs and is now chief technology officer for the company. About the diameter of a dime, each flex sensor stands on eight small, leg-like prongs. Gentle pressure to the top of the sensor causes the whole structure to flatten as the prongs spread out, pushing hair aside and making contact with the scalp for high-resolution EEG recordings.
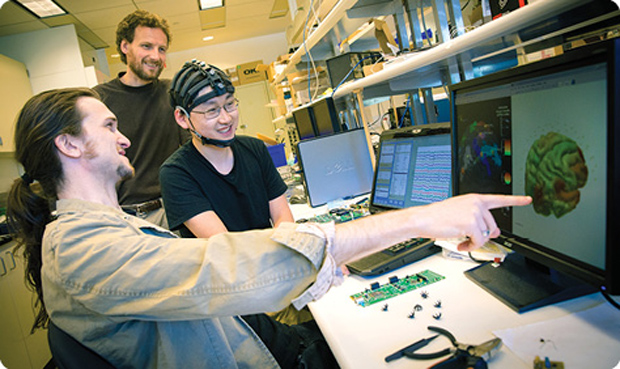
The company also refined the amplification and the shielding to reject environmental interference, the unwanted signals from other nearby devices, such as a power outlet that can contaminate the tiny voltage coming from the body.
The market for the currently available system is mostly university researchers, Chi says. “EEG has been used for a long time, but, for the most part, it’s been constrained to the laboratory. For the first time, researchers can take high-density EEG systems into the real world, where they can perform more realistic neuroscience experiments and collect data in homes, public settings, and other places they weren’t able to before.”
From Soldier to Civilian
Wearable Sensing’s three EEG headsets are research-grade, wireless headsets with either 24 sensors (DSI-24) or seven sensors (DSI-7 and DSI-7-Flex). They utilize noncontact sensors initially developed by QUASAR for military body armor that would monitor cardiac signals and send out an alert if the soldier was shot, including the location of the injury and whether the soldier was still moving or was down on the ground, which could help determine what kind of rescue mission to perform, explains Walid Soussou, vice president of research for QUASAR and president of Wearable Sensing.
![Wearable Sensing’s active dry EEG sensors [shown (a) on the headset and (b) in a close-up] have patented spring-loading mechanisms to mitigate motion artifacts, as well as active and passive shielding. (Photos courtesy of Wearable Sensing.)](https://www.embs.org/wp-content/uploads/2015/03/mertz05-2386451.png)
QUASAR used a similar noncontact sensor technology to design automobile seats that could keep tabs on driver fatigue or other health markers, and firefighter clothing that could measure signs of cardiac distress. Using that technology, Soussou adds, a separate third-party company is now seeking approval from the U.S. Food and Drug Administration for a medical, wearable belt that analyzes biometrics of cardiac health and provides the information to a physician for use during the patient’s next office visit.
Following the cardiac work, QUASAR engineers approached the military about brain monitoring, and the military responded with a long wish list. Some of the requests were that the system be noninvasive and easy to use, yield a high signal quality regardless of environment, be wireless and ambulatory, fit under a helmet, require low power, and, perhaps most importantly, allow easy interpretation of EEG data by nonmedical personnel.
“We did all that and ended up with proof of concept for the first dry-electrode, EEG-monitoring system that produces uncompromising signal quality and low artifacts (environmental interference),” Soussou says (pictured right). He and several other engineers at QUASAR immediately saw the commercial potential of the device and started Wearable Sensing to take it to market. Within a few months of its official start in 2013, the company began offering the three systems for scientific use. “These are super-high-quality, research-grade sensors for people who are doing neurofeedback, neuromarketing, or neuroergonomics work and for researchers who are interested in developing new health monitors,” he says.
When developing the systems, engineers paid special attention to several aspects, including the transmission of data from the headset to a device, whether that is a computer or a smartphone, or to the cloud. “We have positioned our technology to communicate via Bluetooth, so inside each of our headsets is a fully integrated system that has all the amplifiers, the digitizers, the electronics, and a Bluetooth chip,” Soussou says. The company is working with a number of software developers to provide its customers with the communication protocol—a software development kit or an application programming interface—so they can then do further processing of the data as they wish.
In addition, the company developed an algorithm of its own to decode brain activity and provide measures of mental workload, such as engagement and fatigue, in real time and on a personalized level, Soussou says. “If you want to create systems that are reliable, they need to be able to tailor themselves to each individual. That’s where we spend a lot of our time and energy: creating tools that are able to very quickly learn about an individual, so that they know what a high mental workload looks like for that person and can give a very reliable assessment of his or her mental workload.”
Form and Function
One of the biggest challenges for all of these technologies is making the devices comfortable and easy for the users to wear, Chi says. “Coming up with the form factor and mechanics involved years of effort, combining careful design and a lot of trial and error as well as testing on different sized and shaped heads. It’s differences in head shape that makes this a hard problem—and the fact that, unlike many areas of engineering, there are virtually no established, systematic design methodologies.” He adds, “Accommodating human and real-world variability is one of the toughest—yet most important—problems with wearable sensors.”
Wearable Sensing engineers also put a lot of thought into wearability, adds Soussou. “We had to come up with a mechanical structure that could hold the electrodes in place, reliably and reproducibly put them in the right location, and still be very comfortable to wear for a full day.”
The drive toward comfort is especially evident in the work of Vijay Varadan, founder, inventor, and chief medical officer at Nanowear Inc., New York City. He also holds joint appointments as a professor of neurosurgery, engineering science, and electrical engineering at Penn State and as 21st Century Endowed Chair of Nano- and Biotechnologies and Medicine, Distinguished Professor of Electrical Engineering and Biomedical Engineering at the University of Arkansas.
With researchers at both universities and now Nanowear, he is involved in creating an entire line of smart textiles that include consumer-oriented underclothes, socks, and sheets. It all started, however, with the e-Bra (Figure 7). This garment is fitted with printable electronics as well as textile-based nanosensors and nanoelectrodes printed right onto the fabric. Continuously, and in real time, the sensors track blood pressure, respiration rate and oxygen consumption, body temperature, and such electrocardiogram (ECG) readings as inverted T waves, which are a sign of an impending heart attack.

A lightweight, wireless module snaps onto the e-Bra and transmits the information to a smartphone (Figure 8), a Wi-Fi-connected device, or the cloud, or via a digital mobile telephone system (Global System for Mobile Communications), which is used in parts of the world where Wi-Fi is unavailable. In the case of alarming readings or a medical emergency, the system would alert the patient and/or an identified doctor or cardiologist so medical care could begin as soon as possible. For underprivileged locations, he is also instituting mobile clinics through his nonprofit organization, the Global Institute of Nanotechnology for Healthcare. The mobile clinics are designed to pick up alert signals from the system and coordinate emergency care.

The point of the e-Bra was to provide continuous monitoring of women through the clothing that they wear everyday. “It is important because a heart attack can happen anytime, any minute, anywhere,” Varadan says.
From the e-Bra, the work on smart textiles is extending to include a men’s vest and undershirt, a women’s blouse, and bed sheets, all of which are designed to pick up the same measurements as the e-Bra. These products are all in development, and he expects most to become available commercially within about a year.
Another one of Varadan’s projects that is undergoing real-world testing is smart textiles for football players. A football player normally wears a tight-fitting undershirt beneath the shoulder pads, so the researchers wove the sensors into the undershirt and added a wireless communication module to the pads. This system doesn’t need cell phone reception or Wi-Fi, which may not be available in a stadium, and instead uses a Zigbee wireless system, which can easily transmit information from each player’s system to the box office. “This system can pick up ECG to monitor for risk of cardiac arrest,” he says. When it is combined with skullcaps that can pick up EEG measurements and with accelerometers to recognize hard hits to the head, it also has the potential to serve as a concussion alert system.
That’s not all: Varadan’s research group is also developing smart socks that will keep a close watch on blood flow. This would be useful for diabetics, who can have problems with infection stemming from poor circulation in the feet. He expects the socks to also make a commercial appearance in about a year.
The core technology for all of the products is the textile sensor. “We went through about two to three years of work to see how we could make it flexible and comfortable and, at the same time, didn’t lose signal,” Varadan explains. One of the biggest issues was the motion artifact caused as people moved while wearing the smart textiles, he says. “Some other systems deal with the artifacts by simply throwing out those data. For us, though, all of the data is very important, so we ended up taking care of motion artifacts in the software.”
In all, he says, the project involves a combination of many technologies: textile-based nanosensors, printable electronics for communications, and software. “We are now putting all three processes together to make systems that are comfortable and effective.”
Coming Attractions and Challenges
Everyone seems to agree that wearable sensors and smart textiles will become more prevalent, and they’ll have growing health care applications, but some advances will need to be made before the surge really gets going.
One is to get the systems to run on very low power, nearer to the miniscule amount of power the brain draws, according to Cauwenberghs. “Look at the brain with, say, a trillion neurons all working a quadrillion operations per second. A supercomputer that does a comparable task may operate at hundreds of kilowatts, if not a megawatt, of power, whereas the brain does it with just 15 W of power.” Taking inspiration from the brain’s feat, his lab is working on extremely low-power devices that directly interface with the brain.
This will also be important as the demand for wireless capability rises. “Wireless is great, but there is also a mounting awareness that as we add more of these gadgets to the body through a wearable line of sensors, we need to assess the effects of radiation,” Cauwenberghs notes. “Increasingly, we are seeing some evidence that radio-frequency exposure—at levels assumed safe by the federal guidelines—is affecting brain health and activity to a potentially dangerous degree.” He sees this as motivation for scientists and engineers to make devices that yield a minimal electromagnetic footprint. “I think as we build more useful electronic interfaces to the body, we want to make sure that we don’t burden the body with more electricity than needed, especially when they’re used on patients who are already under stress. That’s a challenge that I think we’re beginning to address through better micropower technologies that can lessen the levels of exposure.”
Other areas that still need more work include sorting through and making sense of all of the information—the big data (see “Wearable Tech and Big Data Take on Parkinson’s Disease”)—that wearable sensors and smart textiles will collect and using cloud processing to a greater capacity, Soussou notes. “I think both of these will happen in parallel and will feed one into the other over the next couple of years.”
[accordion title=”Wearable Tech and Big Data Take on Parkinson’s Disease”]
A major collaboration has brought two giants together to fight Parkinson’s disease, a progressive neurodegenerative disorder that affects 7–10 million people worldwide. One player is the Michael J. Fox Foundation for Parkinson’s Research, which describes itself as the largest nonprofit funder of Parkinson’s research worldwide, and the other is global computing pioneer Intel.
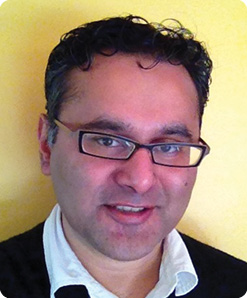
The two are collaborating on a platform that uses data collected through a wearable device and a smartphone to quantitatively measure the diverse symptoms of the disease, such as tremor, gait, or balance disturbances; changes in sleep patterns; and slowness of movement. The idea is to gather information from multitudes of patients and tap the amassed data to create a statistically valid and scientifically useful model of how the disease progresses and, potentially, how medical treatments can improve patients’ quality of life, explains Vin Sharma (right), big data analytics strategist at Intel and a member of the team working on the platform.
“The challenge here was twofold: 1) to be able to collect these observations about the progression of the disease more objectively than from patient reports (as is the standard today) and 2) to be able to do this at a scale where if we did hypothetically have a million patients all providing their data to a shared infrastructure where it could be correlated and analyzed, then researchers and doctors would be able to use it to find patterns, to make predictions, and to understand better the progression of the disease,” he says.
To do it, the researchers turned to available technology, specifically accelerometer- and biosensor-equipped watches that were already on the market. “We then developed open application programming interfaces to access the data in the watch and correlate them with those on a smartphone that’s been carried by the patient,” Sharma explains. Patients wear the watch day and night, and the gathered data provide a time line of changes in their movement and in their sleep patterns. “We also created a small app that runs on the phone and lets the patient annotate their state of mind or whether they took a medication at a certain time. This provides additional user-generated data to relate to the sensor-generated data.”
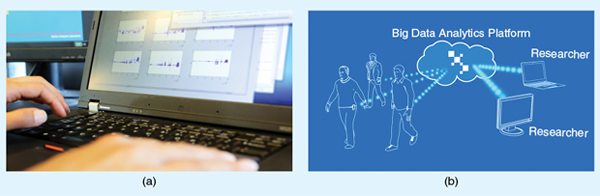
From there, the data are deployed in streaming fashion to the cloud, where a big-data platform awaits (Figure S2). “As the population of monitored patients grows, we anticipate that we’ll eventually need to scale the platform, which will collect, store, process, and allow researchers to analyze the data,” he says, noting that the platform only looks at population data, so patients are completely anonymous. They structured the platform so that researchers can scan across the population for various factors that they believe may be influential in the progression of the disease. The platform also uses machinelearning techniques to search for clusters of data points on its own. “Data-driven discovery is what the researchers are after, because they need data to validate hypotheses, and at the same time, this huge amount of data can help us discover things we wouldn’t have thought of otherwise,” Sharma asserts.
Today, the platform is up and running on a secure, researchers-only site, and a small population of patients from three hospitals in the United States and Israel are already submitting data, primarily as a means to validate the device-to-cloud platform. As work progresses in 2015, the research group is working with the Michael J. Fox Foundation to recruit more patients as well as a control group that will help clarify the model.
Other diseases could also be monitored and analyzed in this manner. Sharma remarks, “Being able to monitor heart rate and look for patterns that correlate with other data points could be very fruitful for cardiovascular applications in medical research and/or treatment for heart disease, which is one of the most significant and pervasive diseases in the Americas as well as the worldwide population.”
The objective for heart disease is the same as for Parkinson’s, he notes. “We want to get more objective measurements from individual patients and also look for patterns across the population of patients to ultimately improve the quality of care that the patient receives.”
[/accordion]
Advances like these will turn the prospect of wearable biomedical sensors into a reality, and that will have impacts in research, medicine, and consumer usage. Soussou adds, “The ability to monitor ourselves individually and ourselves in the world is something that wasn’t available before. Now everybody from kids to scientists are getting more and more of these tools and are not only starting to find answers to questions but are finding new questions to ask.”
References
- Y. M. Chi, T. P. Jung, and G. Cauwenberghs, “Dry-contact and noncontact biopotential electrodes: methodological review,” IEEE Rev. Biomed. Eng., vol. 3, pp. 106–119, 2010.
- S. Ha, C. Kim, Y. M. Chi, A. Akinin, C. Maier, A. Ueno, and G. Cauwenberghs, “Integrated circuits and electrode interfaces for noninvasive physiological monitoring,” IEEE Trans. Biomed. Eng., vol. 61, no. 5, pp. 1522–1537, 2014.