When brothers Jamie and Glen Selby, aged 5 and 7, arrived at the Shriners Burns Institute in Denver, Colorado, in July 1983, more than 97% of their skin had been destroyed by a fire they had accidentally started while playing in an abandoned house. The boys were so badly burned that their outlook was grim—a 6-year-old friend who was also in the fire died from his injuries—but Jamie and Glen were lucky. Not only did they survive, but they were also some of the first patients to benefit from a new burn treatment nicknamed test-tube skin.
Autografting—taking samples of healthy skin from a patient and grafting them to the burn site—is the standard treatment for severe burns, but sometimes there simply isn’t enough healthy skin to cover the complete burn wound. Test-tube skin changed everything. Massachusetts Institute of Technology researcher Howard Green unexpectedly discovered that he could regenerate skin cells when he failed to create a model of a rare type of mouse tumor, according to his New York Times obituary (Green died last year). Green used this technique on the Selby boys, taking postage-stamp-sized squares of healthy skin from them and putting these in a special solution that allowed epithelial stem cells in the skin to replicate 10,000 fold. This expanded skin was then grafted onto the boys’ wounds. The technique was lifesaving.
As Green told a reporter from the Times at the time, “We weren’t prepared to do it on that scale because we did not have the trained manpower, but we agreed to try because the boys had no chance to survive otherwise.” The new skin successfully integrated into the boys’ bodies and began to function normally. Jamie and Glen returned home after about a year in the hospital.
This test-tube skin, the first therapeutic use of cultured cells, gave new hope to victims of third-degree burns and opened the door to the development of stem cells as treatments for burns and other medical maladies. Now, more than 30 years later, researchers are building on this discovery to create new methods for treating burn wounds, including innovations— spray-on skin, three-dimensional (3-D) printed skin, pulsed electric field treatment, and epidermal electronics—that sound like science fiction.
Spray-On Skin
While test-tube skin can help when there’s not enough donor skin, its disadvantage is that it can take weeks to grow the skin cells in the lab. This delay means lengthy hospital stays and increased risk of infection and scarring. A new technology known as spray-on skin promises to speed up the process and reduce risks. Using a small biopsy of a patient’s healthy skin, Avita Medical’s ReCell device (Figure 1) creates what the U.K.-based company calls Regenerative Epithelial Suspension (RES). Within about 30 minutes, the RES, which contains cells and wound-healing factors from the patient, can be sprayed onto the burn wound, covering an area up to 80 times the size of the biopsy site. The system can be used alone to replace the epidermis, but for reconstructing the lower dermis layer in patients with deep burns, it must be used in conjunction with other technology.
A study of 82 patients compared ReCell’s spray-on skin to traditional skin grafts in treating deep partial-thickness burns and found that the spray treatment was both faster and less painful [1]. Plus, the healed skin resulting from both treatments looked and functioned the same. And a more recent, smaller study found that patients who had tried both the spray-on skin and a traditional graft were more satisfied with the spray-on skin because the donor site was considerably smaller and less painful [2].
In the United States, Avita Medical is currently recruiting participants for a stage-two clinical trial. In December 2015, the U.S. Food and Drug Administration (FDA) granted the treatment an Expedited Access Pathway designation, which may speed up its regulatory approval. Under the FDA’s Compassionate Use program, it is currently approved for use in U.S. burn centers to treat patients who have no other treatment options. The system is already approved for use in many other countries.
3-D Skin Printer
A 3-D skin printer may sound improbable or futuristic, but that’s just what an interdisciplinary team at the University of Toronto’s Sunnybrook Research Institute is working on—with the goal of creating the next generation of skin substitutes for burn patients. The group includes Marc Jeschke, M.D., Ph.D., a clinical scientist who works with burn patients; microfluidic scientist Axel Guenther, Ph.D.; and stem cell biologist Saeid Amini-Nik, M.D., Ph.D.
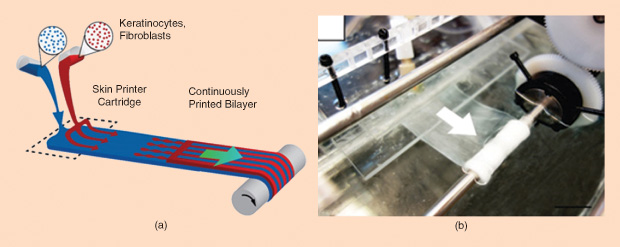
The process begins with stem cells that are harvested from a patient, then turned into specific types of skin cells, and multiplied in the lab. The printer precisely determines the spatial location of each cell, combining them with a gel-like scaffolding substance. The result: strips of substitute skin (Figure 2). “The main thing is that, for burn patients, we need to manufacture a skin substitute that has a dermis component plus an epidermal component,” explains Amini-Nik.
The group has successfully printed a two-layer skin substitute made up of human keratinocytes, the main component of the top epidermal layer of skin, and fibroblasts, the main component of the lower dermis layer. And they have experimented with grafting the printed skin onto a mouse with a compromised immune system (used so the mouse wouldn’t reject the human stem cells). The result? According to Amini- Nik, “We saw that after seven to ten days, the skin healing really improved.”
Before moving on to testing the printed skin on pigs or humans, the group is refining the technology to make the best possible skin substitute. For instance, the original printed skin was quasisolid when it came out of the printer; now, the group is testing printing technology that allows the skin to come out fully solid, making it easier to handle. The group plans to continue developing this technology to make the printed skin stronger. “At the end of the day, we want to give it to all the surgeons in the world so they can print skin substitute and put it on patients,” says Amini-Nik. “They need something stronger.” The team is also testing the possibility of grafting substitute skin containing stem cells directly onto the body rather than waiting for these cells to differentiate into skin cells in a dish. All in all, the group is very hopeful about this technology. “We’ve started preparing our protocols for clinical trials,” Amini-Nik continues, “because we know how much patients are in need.”
Printed skin could be especially helpful for patients with severe burns that cover large portions of their bodies. As Amini- Nik explains, “We lose patients because of this—there’s not enough skin.” Because the stem cells used in printed skin could come from organs other than skin, such as bone marrow, patients would be able to have a skin substitute that contains their own cells. Moreover, according to Amini-Nik, the skin printing technique can be economical in its use of stem cells because it can print islands of cells that will then grow to fill in the space between the islands: “Imagine a patient has 60% of [his or her] skin gone, and then you isolate some stem cells, but you don’t have a lot of them. Then you need a technology that can—especially for epidermal cells—put them close together because they can grow and differentiate better compared to random distribution.”
An exciting possible extension of this work—although it would be far in the future at this point—is a printer that could theoretically integrate the stem cells that give rise to accessory organs, like sweat glands or hair, into the printed skin substitute.
Preventing Burn Scars
Indeed, one reason burns are particularly difficult to treat is because they destroy more than just skin cells. “You are certainly destroying cells, but you are also destroying the extracellular matrix around the cells, the conduits that carry blood via small arterioles, venules or capillaries, and nerves,” explains Martin Yarmush, M.D., Ph.D., who directs the Center for Engineering in Medicine at Massachusetts General Hospital. “Burned tissue is basically overcooked meat.”
Yarmush and his group have demonstrated that a technique using pulsed electric fields (PEFs) may be helpful in healing burns and reducing scarring because it can destroy only cells, which can regenerate, while leaving the cells’ support structures intact [3]. Many burn patients suffer from hypertrophic scars, which are raised, red, and often itchy. These scars contain excessive collagen and may restrict movement. Yarmush and his team have found that applying short bursts of high-voltage PEFs to a healing burn wound can promote a redirection of the healing process and reduce scar formation, as shown in Figure 3(a).
The technique works because the PEFs create holes in the membranes of cells, allowing the cells’ internal contents to leak out. Importantly, PEF treatment leaves other structures—like the extracellular matrix and the basic organization and structures of sweat glands, arteries, veins, and hair follicles—intact. In fact, Yarmush’s group has observed the regrowth of accessory organs following PEF treatment of normal rat skin, regrowth that could have important clinical applications down the line.
“A major challenge in burn injury is if [patients are] burned over a major part of their body and the surgeon uses artificial skin to cover this large area, these patients will lack accessory organs— such as sweat glands—over a major portion of their body,” says Yarmush. He adds that “patients with major body surface area burns must be in air conditioned rooms in the heat because without sweat glands they have difficulty releasing internal heat.” Yarmush calls the ability to regrow accessory organs one of the holy grails of skin tissue engineering, although he’s quick to point out that this potential application for PEF is now only theoretical.
Yarmush’s group has also shown via experiments in mice that PEF treatment can disinfect burn wounds by killing microbes in the wound [4], as shown in Figure 3(b). In fact, the technology has its roots in the food industry, where it is used to get rid of pathogens like salmonella. A major advantage of this technique is that—because it is purely physical—it can kill antibiotic-resistant bacteria. It can also be used in conjunction with drugs because its ability to punch holes in cell membranes could, theoretically, increase drug penetration into bacterial cells.
Although PEF technology for another application—tumor ablation—has already been shown as safe in human clinical trials, its applications for treating burn wounds are still in the animal-testing phase. Yarmush and his group are also exploring whether the technique can be used for other applications such as hair regrowth following chemotherapy or skin rejuvenation. And they are teaming up with a lab at Tufts University to examine the possibility of developing flexible electronics that can deliver PEFs. “Today we utilize hard electrodes where we lift the skin and place it in between two metal electrode plates,” Yarmush says. “Our question is: can we apply PEFs with flexible electronics which are eventually wearable?”
Monitoring Healing
Flexible electronics are the name of the game for Northwestern University professor John A. Rogers, Ph.D. His group has developed a series of epidermal electronic sensors [5] that can be used to monitor the health of skin, including the healing of skin wounds following burns or surgery (Figure 4). “These are devices that have kind of the properties of skin,” says Rogers. “They’re super-thin and soft and can—in a nonirritating, noninvasive way—laminate right onto the surface of the skin.” These devices can make a range of different measurements useful for clinicians who want to know how successfully a wound is healing: they can provide information about inflammation and possible infection via high-precision spatial temperature mapping, indicate how hydrated the skin is by measuring thermal conductivity, and measure blood flow to monitor revascularization. “We can do all of those different things in a single platform,” Rogers explains.
Rogers’ group has demonstrated how effectively these devices monitor surgical-site healing in human clinical trials, and it is now focusing on using them to monitor how well skin grafts are being integrated into the body. “I’m told that a key part of the healing process involves the establishment of microvascular blood flow up through the skin graft from the surface of the burn site,” notes Rogers. “Our devices can measure that.”
While Rogers has plans to test chronic integration of these devices into animals, the current models are disposable, similar to a bandage. They can be used just about anywhere on the body—even on the most fragile skin, such as that below the base of the eye lid or on the textured surface of an ear. These wearable electronics are much thinner than a sheet of paper. Rogers likens them to a temporary tattoo. “It has a very slight tackiness,” he says. And, theoretically, epidermal electronics could come in any size. “One of the key features of the technology is the way that we fabricate them leverages the semiconducting processing approaches that are used for conventional electronics. So it’s intrinsically then scalable to very, very tiny dimensions at the level of the individual sensors and up to very large areas in terms of the overall size.”
A big advantage of these flexible epidermal electronics is that they are so noninvasive. Other instruments currently used to collect similar data are hard, handheld devices that are pressed against the skin and can’t be used on the most sensitive areas, such as the face. In fact, they can actually change the parameter being measured due to the force required to collect data. And because these new epidermal electronics are so noninvasive, people may be more willing to try them out. The devices, says Rogers, are “not threatening in the same way that a scalpel or a hypodermic needle or even a stethoscope is—all these kinds of hard, cold metallic tools.”
In the future, epidermal electronics may also come imbedded with technology that could stimulate the skin, provide thermal therapy, or deliver drugs. “We have electrically controlled pumps that allow us to pump pharmaceutical agents out of a reservoir down into the depths of the brain, but I think that same technology capability can be imbedded in these skin-mounted patches,” Rogers contends. “I think it’s a really interesting direction for the future, and we’re excited about that.”
References
- G. Gravante, M. D. Fede, A. Araco, M. Grimaldi, B. D. Angelis, A. Arpino, V. Cervelli, and A. Montone, “A randomized trial comparing ReCell system of epidermal cells delivery versus classic skin grafts for the treatment of deep partial thickness burns,” Burns, vol. 33, no. 8, pp. 966–972, 2007.
- R. Sood, D. E. Roggy, M. J. Zieger, M. Nazim, B. C. Hartman, and J. T. Gibbs, “A comparative study of spray keratinocytes and autologous meshed split-thickness skin graft in the treatment of acute burn injuries,” Wounds, vol. 27, no. 2, pp. 31–40, 2015.
- A. Golberg, G. F. Broelsch, S. Bohr, M. C. Mihm, W. G. Austen, H. Albadawi, M. T. Watkins, and M. L. Yarmush, “Non-thermal, pulsed electric field cell ablation: A novel tool for regenerative medicine and scarless skin regeneration,” Technology, vol. 1, no. 1, pp. 1–7, 2013.
- A. Golberg, G. F. Broelsch, D. Vecchio, S. Khan, M. R. Hamblin, W. G. Austen, R. L. Sheridan, and M. L. Yarmush, “Pulsed electric fields for burn wound disinfection in a murine model,” J. Burn Care and Res., vol. 36, no. 1, pp. 7–13, 2015.
- D. H. Kim, N. Lu, R. Ma, Y. S. Kim, R. H. Kim, S. Wang, J. Wu, S. M. Won, H. Tao, A. Islam, K. J. Yu, T. Kim, R. Chowdhury, M. Ying, L. Xu, M. Li, H. J. Chung, H. Keum, M. McCormick, P. Liu, Y. W. Zhang, F. G. Omenetto, Y. Huang, T. Coleman, and J. A. Rogers, “Epidermal electronics,” Science, vol. 333, pp. 838–843, 2011.