Over the past decade, embedded systems and microelectromechanical systems have evolved in a radical way, redefining our standard of living and enhancing the quality of life. Health care, among various other fields, has benefited vastly from this technological development. The concept of using sensors for health care purposes originated in the late 1980s when sensors were developed to measure certain physiological parameters associated with the human body. In traditional sensor nodes, the signal sources are mostly different environmental phenomena (such as temperature, vibration, and luminosity) or man-made events (such as intrusion and mobile target tracking), whereas in case of the physiological sensors, the signal source is living human tissue. These sensor nodes, as their primary sensing element, have a diaphragm that converts pressure into displacement. This displacement, in turn, is subsequently transformed into an electrical signal.
The concept of wireless physiological sensor nodes, however, gained popularity in the mid-2000s, with the sensed data from the nodes transmitted to the hub via a wireless medium. The network formed by this heterogeneous set of wireless body sensor nodes is termed a wireless body-area network (WBAN). Each WBAN is essentially a composition of multiple wireless body sensor nodes and a single hub. The hub is primarily responsible for acquisition of the raw sensed data from all the component sensor nodes and first-level aggregation of the data before transmitting the aggregated data for further analysis to a remote data acquisition center. Here, we outline the evolution of WBANs in the context of modern health care and its convergence with nanotechnology.
Enhancing Traditional Health Care FAcilities
Conventional health care amenities demand direct patient–doctor interaction, where the doctor physically checks the patients and treats them accordingly. This policy, however, is highly inconvenient for patients suffering from chronic diseases, postoperative health monitoring, and activity monitoring in the elderly. In this context, it is noteworthy that, according to recent calculations by demographers, the global elderly population (over 65 years old) reached about 760 million in 2015—almost doubling from what it was in 1990. This increasing elderly population suggests that by 2050, elderly health monitoring will be a critical issue posing serious challenges to conventional medical practice and technology. WBANs could provide seamless, ubiquitous, and remote health care and, therefore, prove to be highly effective in this context. The wireless body sensor nodes are miniaturized in size—composed of several micro-order components. These sensors are planted on the patient’s body, and the patient is allowed to move and perform his or her regular activities while being monitored on a continuous basis. Patients suffering from chronic diseases, such as heart and lung diseases, demand long-term periodic follow-ups, and use of wireless heart rate and electrocardiogram (EKG) sensors allows for the real-time analysis of the patients’ condition. The network architecture of WBAN-based medical care in a hospital environment [1] is shown in Figure 1. Wireless accelerometer sensors help in fall detection for elderly people living alone.
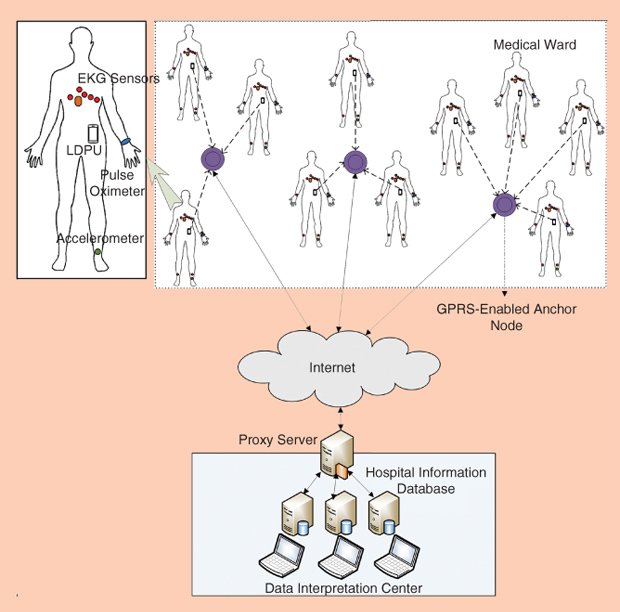
The use of WBANs is also extremely relevant for emergency health care. The wireless sensor nodes are usually integrated into clothing (in the form of a vest or band), which the patient is made to wear; in some cases, however, the sensors are simply patched onto the patient’s skin with assistance from a paramedic immediately after the patient is aboard an ambulance. The real-time physiological data of the patient are then transferred to a remote hospital where doctors and medical experts monitor his or her vital parameters, and, based on the chief complaints and the medical history of the patient, immediate medication and treatment instructions are sent to the paramedic. Also, this helps the doctors to have a priori information about the patient’s status so that necessary treatment can be started upon the patient’s arrival at the hospital without any time loss. Thus, these lightweight, miniaturized, and portable sensor devices are shaping modern health care and gradually yet constantly mitigating the ageold inconveniences and clichés associated with conventional health care practices.
Applications of WBANs
A WBAN is a collection of heterogeneous sensor nodes that are linked to a single hub acting as the local data aggregation and processing unit. A hub can be conceptualized as a portable device such as a simple smart mobile phone, a tablet, or a personal digital assistant, which is capable of connecting to the Internet. Based on the placement of the wireless sensor nodes, WBANs are classified into two broad categories: 1) onbody wearable WBANs and 2) implantable WBANs. Long-term health monitoring of chronic patients, smart hospital-based patient treatment, ambulatory health care, assessment of soldier health, and fall detection for elderly people are a few of the many applications of on-body wearable WBANs. Figure 2 shows the Shimmer WBAN setup and a patient wearing the EKG and pulse oximeter sensors.
In certain cases, however, the tiny wireless sensor nodes may be implanted within human tissues or in the blood stream. These physiological sensor nodes are specially designed to be ultralightweight (less than a gram) and miniaturized (less than a few square millimeters). These nodes have an extremely low power consumption rate and usually have a data rate of a few kilobits per second. The implanted WBANs are envisioned to be used in situations demanding high precision in continuous measurement of certain invasive physiological properties or typical applications where on-body sensors are inapplicable. For example, patients with diabetes require their blood sugar level to be monitored on a continuous basis and with precision. An implantable glucometer would be highly effective in such a case. Implantable WBANs could also assist in advanced medical services, such as detection of cancerous cells within the body and improving eyesight for people with vision impairment.
Apart from the aforementioned applications, WBANs also support nonmedical applications, such as real-time audio and video streaming, emotion detection, biometric authentication, and detection of nonmedical emergencies (e.g., the presence of poisonous or inflammable gas and fires).
Communication Standard
Initially, the WBAN-based communication took place in either of the two communication standards—IEEE 802.15.1 and IEEE 802.15.4. The IEEE 802.15.1 standard, popularly known as Bluetooth, operates at a data rate of 3 Mb/second and has a communication range of 1–100 m, as shown in Table 1. However, the response time for devices operating on Bluetooth is fairly high, and, more importantly, the Bluetooth devices consume a considerably high amount of energy, demanding frequent recharging of the batteries. In such a context, the IEEE 802.15.4 standard, also known as the ZigBee standard, is considered to be more suitable to support WBAN-based communications. IEEE 802.15.4 was designed specifically for wireless personal area networks supporting longrange communication with a low data rate of up to 256 kb/second. However, while WBAN-based communication does not require long-range data transmission (generally limited to only a couple of meters), it requires higher network bandwidth for smooth traffic flow without incurring any unnecessary delay. WBAN-centric communications are also expected to be highly energy efficient for longer usability (lifetime) of the body sensor nodes.
[accordion title=”Table 1. Comparative Studies of Different IEEE Standards”]
IEEE Standard | Operating Frequency | Data Rate | Range (m) |
---|---|---|---|
802.15.1 | 2.4 GHz | 1 Mb/second | 1-100a |
802.15.4 | 868 MHz, 915 MHz, and 2.4 GHz |
20-250 kb/second | ~75 |
802.15.6 | 3993.6 MHzb and 7987.2 MHzc |
1 Gb/second | 10 |
aBluetooth radio—Class 3: 1 m; Class 2: 10 m; Class 1: 100m.
bUltrawideband (UWB) mandatory operating frequency: Low band—3993.6 MHz.
cUWB mandatory operating frequency: High band—7987.2 MHz.
[/accordion]
Therefore, in 2007, the IEEE formed a new task group to constitute a new standard specifically to support WBAN-based communication by supporting its requirements, and, in 2012, the IEEE 802.15.6 standard was published. The IEEE 802.15.6 standard proposes a new set of physical layer and medium access control sublayer protocols while complying with the medical guidelines. It provides a set of guidelines for ultralow-power, short-range (within 10 m) communication with high data rates (up to 10 Mb/second) in the vicinity of or within the human body. With support for high payload size (maximum of 256 B), the standard guarantees a packet error rate of less than 10%, ensuring high reliability in packet transmission. A WBAN operating on the standard is capable of adding or removing a node from the network in less than 3 seconds without introducing any significant delay. The transmission latencies for medical and nonmedical applications are expected to be less than 125 and 250 milliseconds, respectively. The IEEE 802.15.6 standard also provides strict noninterference guidelines along with improved quality of service and reliability in communication. The standard clearly mentions that the nodes of a WBAN are connected to a central hub in a single-hop star or a top-hop extended star topology.
A maximum of 64 nodes may be connected to a single hub. Among other notable changes, the IEEE 802.15.6 standard introduces eight different user priorities based on the traffic designation [2], as shown in Table 2. Higher user priority indicates the corresponding data packet has a higher priority for being transmitted, i.e., lower delay is incurred during its transmission. The justification for introducing the eight distinct user priorities is to prioritize the data transmission based on the type of data. While data generated by processes running in the background may be transmitted infrequently after long time intervals, such delays are intolerable for other types of data. Especially for medicaland emergency-event-related data, any sort of undesired delay may result in untimely event reporting or even loss of data. Performance analysis of the standard [2] shows that it successfully prioritizes the transmission of medical- and emergency-event related data and ensures extremely low transmission latency.
[accordion title=”Table 2. User Priorities Based on Traffic Designation”]
User Priorities | Traffic Designation | Priority |
---|---|---|
0 | Background (BK) | Lowest |
1 | Best effort (BE) | |
2 | Excellent effort (EE) | |
3 | Controlled load (CL) | |
4 | Video (VI) | |
5 | Voice (VO) | |
6 | High-priority medical data or network control | |
7 | Emergency or medical event reporting | Highest |
[/accordion]
From Micro- to Nanotechnology
Although micro-ordered body sensor nodes have significantly advanced health care options, these WBANs have restricted applicability under certain constrained situations. The component sensors of a microscaled WBAN are unable to provide absolute invasive medical care and also fail to provide cellular-level precision in measurement and actuation. Invasive health care using these wireless sensors is also understood to be inconvenient as it requires piercing and penetration of the patient’s body. The latest advancements in nanotechnology have provided a suitable solution to the aforementioned limitations of the microscale WBANbased health care. Nanotechnology enables fabrication of devices in the scale of 1 to 100 nm. These nanodevices can be envisioned as a tiny arranged set of molecules able to perform small and simple sensing, actuation, and computation tasks. As proposed by Akyildiz et al., a nanosensor node consists of multiple nanocomponents [3], each designed to perform a particular task.
These nanocomponents, when connected, form a wireless nanobiosensor network, and the sensors communicate with a static or mobile nanoscale data-acquisition module. However, communications among the nanobiosensor nodes do not follow the existing wireless communication protocols. The primary differences in communication involving a traditional sensor network and a nanobiosensor network are the modes and medium of communication. Unlike traditional wireless sensor networks, in nanonetworks, communication between a pair of nodes may take place electromagnetically, mechanically, or molecularly. The most common means of communication within nanonetworks is through electromagnetic waves using nanotransceivers and nanoantennas for high-frequency communication. However, because of the complexity involved in manufacturing the nanotransceiver units, it is quite difficult to integrate several nanoscale components into a nanodevice. Also, the output power of the nanotransceiver would not be strong enough to establish a bidirectional communication channel [3]. On the other hand, mechanical communication involving the nanodevices requires physical contact between the transmitter and receiver units and, therefore, is largely unsuitable because physical contact of the nanobiosensor nodes within the human body is not guaranteed. Molecular communication among nanodevices, however, is considered to be highly likely in the context of in-body communication. In this mode of communication, messages are encoded within the molecules using certain internal molecular parameters, such as chemical structure, relative position of elements, or polarization. Integration of molecular transceiver units on the nanodevices is comparatively more feasible because of its miniaturized size.
Another key difference between the traditional wireless sensor networks and the nanosensor networks is the data transmission speed. In the case of the former, signals are transmitted at the speed of light, whereas in the case of nanobiosensor networks, information transmission is heavily dependent on the mode of communication. For example, in electromagnetic communication mode, data are transferred considerably faster compared to molecular communication mode [3]. The impact of the electromagnetic waves on the human body is also a serious cause of concern as we talk about electromagnetic data transfer with the body. Apart from these, the noise associated with communication occurring within the human body also demands special consideration for classification and separation.
Some of the direct application areas of wireless nanobiosensor networks in the field of medical science include the following.
- Health monitoring: Nanosensors are able to offer precision at the cellular level. To provide a better idea regarding the precision offered by the sensors, note that a nanobiosensor node is able to distinctly measure pH at two different places within a single cell. Measurement of oxygen and cholesterol levels, identification of cancerous cells, and diagnosis of hormonal disorders are a few of the tasks able to be performed by wireless nanobiosensors.
- Drug delivery: Precision drug delivery is another important application of wireless nanobiosensor networks. Smart nanoactuators are used to deliver drugs invasively without external interference. For example, smart insulin nanoactuators can constantly monitor the blood sugar level and inject precalculated amounts of insulin upon determination of insulin deficiency. Other examples include treatment of cancerous cells using cellular-level chemotherapy.
- Immunity support: Special types of nanobiosensor nodes can identify the presence of foreign bodies and pathogens within the human body and are capable of responding against the malicious cells, resulting in disease treatment that is localized, less aggressive, and less invasive.
However, practical implementation of wireless nanobiosensor network-based health care is yet to be realized. Although recent advancements in nanotechnology and nanoscience have triggered the manufacturing of individual nanocomponents, such as nanomemory and nanoantennas, aggregation of all these components to construct a nanosensor node has yet to be achieved. Design and development of nanotransducers, defining a network topology, identification of a suitable communication mode, and creation of efficient encoding and decoding methods for messages are a few of the important current research challenges. However, with the advancements in interdisciplinary research, nanotechnology will extend its applicability and impact on various fields and, in the future, will revolutionize the scientific and technological community and, in turn, human life.
References
- S. Misra and S. Sarkar, “Priority-based time-slot allocation in wireless body area networks during medical emergency situations: an evolutionary game-theoretic perspective,” IEEE J. Biomed. Health Inform., vol. 19, no. 2, pp. 541–548, Mar. 2015.
- S. Sarkar, S. Misra, B. Bandyopadhyay, C. Chakraborty, and M. Obaidat, “Performance analysis of IEEE 802.15.6 MAC protocol under non-ideal channel conditions and saturated traffic regime,” IEEE Trans. Comput., vol. 64, no. 10, pp. 2912–2925, Oct. 2015.
- I. F. Akyildiz, F. Brunetti, and C. Blazquez, “Nanonetworks: a new communication paradigm,” Comput. Networks, vol. 52, no. 12, pp. 2260–2279, Aug. 2008.