In August 2015, Akimasa Hirata, Professor at the Nagoya Institute of Technology in Japan, received a Technical Achievement Award from the IEEE Electromagnetic Compatibility Society for “his contribution to computational dosimetry and standardization for human exposure to electromagnetic fields.” We invited Dr. Hirata to explain more about his work.
Historically, the computational techniques for electromagnetic dosimetry have progressed significantly for human safety as public concerns have grown over the adverse health effects of environmental electromagnetic fields such as those created by power lines, mobile phones, etc. Research coming out of our laboratory at the Nagoya Institute of Technology provides rationale of the metrics and limits for human protection that are set in the international standards/guidelines by IEEE and the International Commission on Non-Ionizing Radiation Protection (ICNIRP), which are mentioned by the World Health Organization.
State-of-art computational modeling becomes a powerful tool when quantifying induced electrical fields in an anatomical human model. The methods developed are applicable not only to human safety but also relevant to medical applications and health care. The feature of our computational code on electromagnetics (for frequencies <100 kHz) is that the computational speed of electromagnetic software based on the multi-grid method is much faster than that of conventional methods [1]. It takes less than 10 sec for one exposure scenario in an anatomical head model with a resolution of 1 mm. This computational code makes it easier to analyze the individual variability of induced fields in individualized models (e.g., [2]).
One application of our electromagnetic software is in the area of neurosurgery. During brain surgery, electromagnetic stimulation is applied to identify which brain regions are critical for patients’ well-being so that those regions can be maintained as much as possible when removing the tumor. It is essential that we work with individualized head models based on medical images to predict brain activation or other dosimetry. Our group has been able to reconstruct realistic head models with a resolution of 0.5 mm from magnetic resonance images (T1 and T2) of patients. The head models are segmented into 10 anatomical tissues. Schematic explanation of the model development can be found in Figure 1, and the detailed procedure for generating head models are described in our previous study [2].
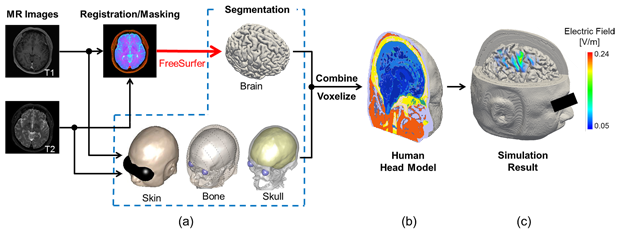
Before neurosurgery, non-invasive diagnosis with transcranial magnetic stimulation (TMS) is conducted. The TMS is a scheme to simulate a target area of the brain by a current induced below a stimulation coil to map cortical regions (e.g., motor and language function). However, that location is mislocalized due to the complicated structure of the brain (e.g., [3, 4]). Thus, the location of the concerned and critical cortical areas must be confirmed by direct electrical stimulation (DES) during brain surgery while the patient is conscious with the information obtained by TMS. The time needed for finding critical cortical areas is substantial (at least a few hours), limiting the number of patients an operating theatre can handle. If we could localize the brain function precisely by TMS preoperatively, the intra-operative cortical mapping to identify the functional localization of the cortex may reduce the time the patient must remain conscious but in the operating room. As shown in Figure 2, the location of the concerned area is well localized using the computational electromagnetics in an individualized head model. The same electromagnetic simulation tool was extended to investigate other treatments, e.g., transcranial or cortical electrical stimulation.
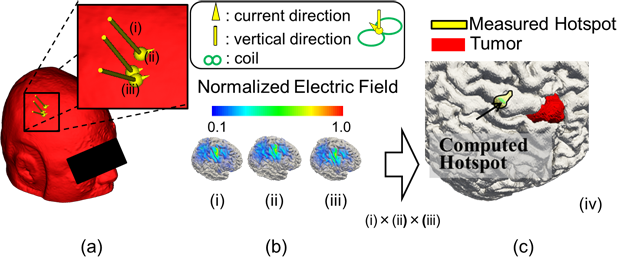
Fast computational software for electromagnetics makes the individualized risk assessment of exposure to electromagnetic fields possible, opening a new avenue for the role of electromagnetics in medicine. The ICNIRP, a partner of World Health Organization, recently published a statement of human safety for medical devices. Computational techniques may soon become important when assessing side effects in diagnosis. As part of this development, we at Nagoya Institute of Technology are currently working on a project that applies machine learning techniques to obtain activation regions by TMS based only on medical images. In the near future, clinicians will be able to make diagnoses solely from medical images without electromagnetic computation; the bottleneck is not the electromagnetics but the segmentation of the medical images.
References
- Laakso and A. Hirata, “Fast multigrid based computation of induced electric field for transcranial magnetic stimulation,” Phys. Med. Biol., vol.57, pp7753-7765, 2012
- I. Laakso, S. Tanaka, S. Koyama, V. De Santis, and A. Hirata, “Inter-subject variability in electric fields of motor cortical tDCS,” Brain Stimulation, vol.8, no.5, pp.906-913, 2015.
- A. Opitz, M. Windhoff, R. M. Heidemann, R. Turner, and A. Thielscher, “How the brain tissue shapes the electric field induced by transcranial magnetic stimulation,” Neuroimage, vol.58, no.3, 849-859, 2011.
- M. Iwahashi, J. Gomez-Tames, I. Laakso, and A. Hirata, “Evaluation method of internal electric field in standardized human brain for different transcranial magnetic stimulation coils,” Phys. Med. Biol., vol.137, 2017 (in press).