We are in the midst of a CRISPR craze. The last five years have seen the publication of over 1,000 scientific papers, the allocation of millions of research dollars, and the establishment of four start-up companies in the United States alone. Internationally, the genome-editing market, fueled by CRISPR technology, is expected to be worth more than US$3,000 million by 2019.
In essence, CRISPR is like a shiny new toy that, for many scientists, would seem to hold all the bright promise and eager anticipation of any unwrapped gift. It may potentially help to eradicate many forms of disease, including painful genetic disorders that have plagued humankind for centuries. It may hold the key to solving numerous public health problems, such as malaria and Lyme disease. Far in the future, in what may seem more science fiction than reality, it may also offer the potential to manipulate human embryos to create designer humans.
The gene-editing technique, derived from a bacterial immune system, allows for the accurate and efficient targeting and modification of specific snippets of DNA. Researchers are already using it to create designer dogs and pet micropigs in China, to concoct new enzymes for industrial uses like laundry detergents and paper milling, and even to make strains of bacteria for producing tastier yogurts. CRISPR is being used in agriculture to make crops hardier and more resistant to pests and drought, as well as to produce livestock with more muscle mass and leaner meat.

The excitement around the technology has led a handful of companies to dedicate themselves fully to CRISPR-based gene therapies. There is Caribou Biosciences in Berkeley, California, along with three companies based in Cambridge, Massachusetts: Editas, Intellia, and CRISPR Therapeutics. Research in the life sciences is quickly moving forward. By 2017, Editas plans to begin testing the technique in treating a rare form of blindness.
“We’re now able to do edits in human cell culture much more easily, and that’s teaching us a lot about ourselves,” says Jacob Corn, managing director and scientific director of the Innovative Genomics Institute at the University of California, Berkeley. “People have also started to show that CRISPR works well in a variety of organisms. The promise there is our understanding of the world around us—the wealth of ways in which biology is regulated and brought together is just going to explode.”
But this potential comes with pitfalls. There are concerns over the long-term ethical implications of such genetic tinkering, even as CRISPR research moves forward at a remarkable pace. Erik Sontheimer, a biologist at the University of Massachusetts Medical School, points to a future in which CRISPR is used to control populations of organisms in the wild, for example.
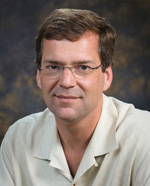
“Gene drives are a CRISPR-based genetic modification that can greatly accelerate the spread of that modification throughout a population,” Sontheimer says. “For instance, if you’ve designed a modification that inactivates a gene important for fertility in mosquitoes and then release a batch of these insects into the wild, you could think about eliminating that population. On one level, that could be useful; maybe these mosquitoes transmit malaria or some other terrible disease. But the question is, what could the unintended consequences be? Can we anticipate everything that might conceivably go wrong? If we can’t, we might not realize it until it’s too late, and the wild population is altered forever.”
From Research to Reality
Although researchers first found CRISPR sequences in Escherichia coli in 1987, it wasn’t until 2012 that a team of scientists led by Jennifer Doudna of the University of California, Berkeley, and Emmanuelle Charpentier of Umea University in Sweden harnessed microbes’ CRISPR/Cas9 system to create a new gene-editing tool. CRISPR is a kind of molecular scissors that can be programmed to snip specific bits of DNA (see “How CRISPR Works”).
[accordion title=”How CRISPR Works”]
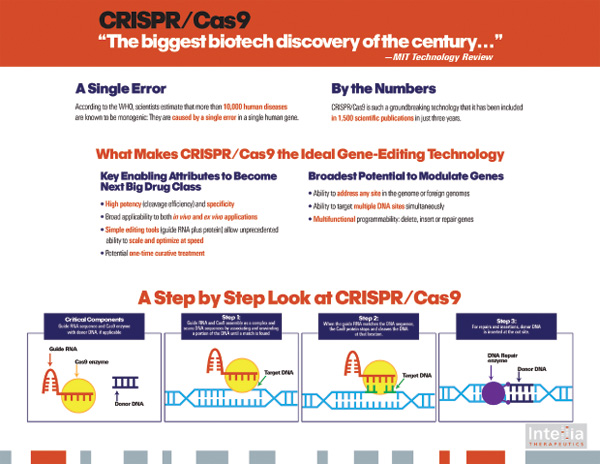
Researchers first found CRISPR sequences in E. coli in 1987, but two decades would go by before they figured out what these sequences did. Scientists observed odd configurations of DNA in bacteria in which a genetic sequence was repeated over and over again, with unique sequences in between the repeats. They named these patterns clustered regularly interspersed short palindromic repeats, or CRISPR. Such CRISPR sequences were subsequently discovered in many microbes.
Researchers determined the purpose of CRISPR when they realized the unique sequences in between the repeats matched the DNA of pathogens that attack bacteria. It turns out that CRISPR is part of an ancient, naturally occurring bacterial immune system. When a bacterium is invaded by a new virus, it copies and incorporates segments of the virus’s DNA into its genome as “spacers” between the short DNA repeats in CRISPR. By keeping bits of these viruses close, the bacterium can recognize and defend against those viruses the next time they attack.
“The CRISPR system allows the bacterial cell to take a little piece of a virus’s genome and record it in its own genome to use as a weapon against the invader,” says Erik Sontheimer of the University of Massachusetts Medical School. “CRISPRs are genetic sequences that are recordings of past invaders that have threatened the ancestors of that cell. It’s like a row of tiny pieces of viral genomes that the cell can access to target those same sequences for destruction if they attack again.”
The CRISPR sequences inserted into the bacterial genome are transcribed and processed into small RNAs. If the RNA molecules recognize an invading sequence of foreign DNA, they guide the CRISPR complex to that sequence. There, a set of enzymes called Cas (CRISPR-associated) proteins recognize and cut the incoming foreign DNA, disabling the invading virus.
Researchers saw the potential for the CRISPR system to cut out selected genes and insert new ones. By delivering the Cas protein and the correct RNAs into a cell, any organism’s genome could be cut at a desired location. It’s an addressable DNA targeting system.

The CRISPR system induces double-stranded breaks in DNA. Those breaks then get repaired in different ways, allowing scientists to disrupt or deactivate genes or change sequences.
CRISPR’s main advantage over its gene-editing predecessors is its ease of use. With other methods like zinc fingers and transcription activator-like effector nucleases (TALENs), scientists had to customdesign an appropriate protein each time—a difficult, expensive, and time-consuming process. You can only target a small number of genes at a time with these methods, and you have to redesign your proteins every time you want to target a different gene.
CRISPR works differently. Rather than relying on proteins, the Cas enzyme uses a guide RNA molecule to find the target DNA, which it then snips. It’s far easier to create an RNA guide sequence than it is to engineer a protein. It’s also much less expensive: zinc fingers can cost upward of US$5,000 to order, while the ingredients for CRISPR can be bought off the shelf for about US$30. This is what makes CRISPR work so easily, and effectively makes it low-tech. You just change the sequence of a small RNA if you want to switch targets from one gene to another.
The elegance and simplicity of the CRISPR technology makes it a viable option for more labs. With CRISPR, scientists can easily manipulate the genes of many organisms beyond the traditional mice and fruit flies. More than three dozen organisms have been genetically edited using CRISPR, including frogs, monkeys, plants, and human cells.
“It has really taken off because you don’t necessarily have to be a gene-editing lab to use it,” says Jacob Corn of the Innovative Genomics Institute at the University of California, Berkeley. “Previous gene-editing technologies were based on proteins recognizing DNA; that means you had to do protein engineering, which worked, but was onerous. The thing about Cas is it’s easy to program and easy to make the reagents. It has really democratized gene editing.”
[/accordion]
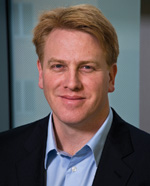
Since 2012, research using CRISPR/Cas9 has exploded. After Doudna, Charpentier, and their colleagues used it as a genome-editing tool in human cell cultures, the technology has been applied to yeast, zebrafish, flies, plants, mice, monkeys, and other organisms. In 2014, Daniel Anderson, a biologist at the Massachusetts Institute of Technology, and his colleagues there demonstrated the first use of CRISPR to fix a disease-causing mutation in an adult animal. The researchers used CRISPR in mice to correct a genetic mutation associated with a human metabolic disease called tyrosinaemia. This is a genetic disease that leads to liver disease and is caused by a single change to the patient’s DNA. In mice with the same mutation, Anderson and his colleagues used CRISPR to correct affected liver cells and treat the disease.
Although it was an important step toward using CRISPR technology for gene therapy in humans, the study also highlighted potential difficulties. For instance, the disease-causing mutation was only corrected in 0.4% of the liver cells.
“The challenge with getting this to work is what we call the delivery challenge,” says Anderson. “In our first study, we used something called high pressure injection, in which you deliver DNA into the bloodstream really quickly and some of the cells in the liver take that DNA up.” This method is generally not considered feasible in human patients. Anderson says he and his team are now working on more patient-friendly ways to deliver CRISPR to affected cells, such as drugs that might be able to deliver the compounds inside patients’ livers.
Real-World CRISPR
CRISPR technology has already moved beyond the lab and into practical applications. Cheese and yogurt makers, such as the DuPont company, have been using CRISPR for nearly a decade to make starter cultures with bacteria that are better able to withstand viral attacks. Scientists in China have used CRISPR to create super-muscular dogs as well as mini pigs that will be sold as pets.
But the biggest buzz is around CRISPR’s potential to treat human diseases. The biotechnology company Editas recently announced plans to start clinical trials in 2017 to treat a rare form of blindness using CRISPR. Editas is focusing on a genetic eye disease called Leber congenital amaurosis (LCA), which affects the light-receiving cells of the retina. Children born with the condition have very poor vision that can progress to complete blindness. It affects only about 600 people in the United States, but Editas is taking on LCA because it will be relatively easy to address with CRISPR. The exact dysfunctional gene, located in the eye’s photoreceptors, is known, and delivering CRISPR machinery to the eye is more straightforward than to many other organs.
Already, scientists at Editas have taken retinal cells from LCA patients and used CRISPR to give those cells proteins that function in cell culture. Further laboratory and animal tests are needed before a human clinical study begins. There are still issues around how well the gene editing will work once in the retina and whether they may be any unintended side effects.

Editas is one of several biotechnology start-ups that are planning to use CRISPR therapeutically. Intellia is a sublicensee of technology from Caribou, which is also an equity holder in Intellia. Thomas Barnes, chief scientific officer of Intellia, says his company has sublicensed the right to make human therapeutics from Caribou, while Caribou retains the rights to agriculture, veterinary tools, and industrial microorganisms.
“Intellia was formed a little over a year ago to pursue these human therapeutic opportunities,” Barnes explains. “We’re exploring ways to deliver this technology. There is in vivo editing, in which we deliver CRISPR inside the body, and there is also ex vivo editing, in which we take cells outside the body, edit them, and then put them back in the body.”
Intellia has a collaboration with international drug company Novartis to use CRISPR for two kinds of treatments: re-engineering patients’ own T cells and hematopoietic stem cells. They are focusing on a specific type of cancer-fighting T cell called a CAR-T to make chemotherapy more effective, while hematopoietic stem cells reprogram bone marrow cells and can be used to correct genetic diseases in the blood. Barnes says Intellia and Novartis are focusing on these two cell types because you can remove them from the patient’s body, edit them, and then return the cells to the patient.
“Compared with other technologies, CRISPR is moving very quickly,” notes Barnes. “In terms of easy things to do with it, it’s the ex vivo editing. But there are edits which are harder to perform. Gene knockouts are easier, as opposed to putting in a gene that’s missing or correcting a base pair change or swapping in a whole chink of DNA.”
CRISPR is still a relatively new technology, and scientists agree that we will see a lot more research on its safety and efficacy. “It’s a new form of therapy,” says Barnes. “From the beginning, Intellia has had a finish line in mind, which is getting a product approved and making it available to patients.”
Ethical Concerns
With the promise of easy genetic editing comes concern over the safety and ethical implications of tinkering with the human genome. These issues were highlighted when scientists in China announced they had used CRISPR to engineer human embryos. Suddenly, it seemed possible to make heritable changes to the human genome.
There are two types of genetic edits: somatic edits, which are changes to adult cells or tissues and are not heritable, and germ line edits, in which changes are made to sperm or egg cells and passed down between generations. Most of the debate has been around germ line editing, says Corn.”The debate has been, should we pursue editing that may have some sort of benefit but is also heritable? In that case, we’re engineering the human species.”
Scientists and ethicists are concerned about using CRISPR in human germ cells for several reasons. Safety is one primary concern. Researchers still cannot predict where and when off-target mutations will occur and what effects these might have. It is important to be certain that CRISPR does not introduce changes elsewhere in the genome before it’s ready for therapeutic use in humans. Some scientists also make the point that replacing a defective gene with a normal one may seem harmless, but the long-term effects may be hard to predict.
There are also ethical considerations involved editing the human genome. Germ line edits are heritable and could have an unpredictable effect on future generations. However, germ line editing could also confer major benefits to millions of people suffering from genetic diseases. CRISPR could potentially eradicate inherited diseases such as cystic fibrosis and Huntington’s disease from family lines altogether. These devastating genetic diseases could be eliminated before a baby is born.
It is also necessary to consider the slippery-slope argument—that CRISPR could be used not only to treat disease but also to genetically enhance humans. The technology could potentially be used to create “designer babies” with whatever genetic traits people might desire. For this reason, many scientists have come together to support a moratorium on such germ line editing.
“What Intellia and other companies firmly believe is that we should confine our activities to somatic gene editing, editing the cells of the body and not the cells of the germ line,” says Barnes.
In April 2015, the U.S. National Institutes of Health stated that it will not fund any use of gene editing technologies in human embryos. Around the same time, a group of leading scientists issued a statement calling for a worldwide moratorium on germ line editing in humans. The authors noted that current knowledge of genes and their interactions is limited and that manipulating one gene in an embryo could have unintended consequences that would then be passed down to future generations. For now, the answers to these scientific and philosophical questions remain open. But the CRISPR boom keeps growing, as researchers use the technology in medicine, agriculture, and basic science.
The future will inevitably include CRISPR, but there are still challenges. One major hurdle is that of delivery, figuring out a way to get the CRISPR machinery into the affected organs and tissues.
“It’s great, it works in the lab, but how do you get to treating patients and making a difference in people’s lives?” asks Barnes. “The primary challenge is getting it into the cell, and the second challenge is making complicated edits once it’s in there.”
Despite the unknowns, there has been furious activity surrounding CRISPR over the last five years, and it shows no signs of letting up. CRISPR is here to stay, and it is revolutionizing gene editing.