Want the real scoop on doing research in space? Ask someone who’s been there. Two astronauts—Joe Kerwin, M.D., who was on the first manned mission to the U.S. Skylab space station, and Jerry Linenger, M.D., Ph.D., who spent nearly five months on the USSR’s space station Mir—recently spent time with IEEE Pulse to provide a unique and candid look at space-based biomedical research over the years, and they shared their perspectives on why such research is vital to future manned missions as well as improved health on Earth.
Joe Kerwin, Skylab Science Pilot, 1973
A year after U.S. President John F. Kennedy announced his intent in 1961 to land a man on the moon, Joe Kerwin earned his wings as a Navy pilot after already having earned his M.D. degree in 1957. Then, in 1965, he joined NASA as a scientist–astronaut and was swept up in the race to the moon.
One of his many contributions was his role as commander of the Apollo Block II test command service module, a land-based simulation of a spacecraft in flight. The module was basically a vacuum chamber on a rotating platform that was bathed in the light of ultrabright lamps to replicate the brutal heat of the sun. As commander of the module, Kerwin was sealed inside with two other astronauts (Vance Brand and Joe Engle) as part of a test to help clear the way for Apollo 7. The module test was a success, and shortly afterward, Apollo 7 carried the first U.S. astronauts into space orbit. Apollo 7 was a stepping-stone to Apollo 11, which set the first human on the moon in 1969.

Kerwin’s most publicized achievement, however, came in 1973 as science pilot for the mission to board the United States’ first space station, Skylab, which had been launched just a few days earlier. Kerwin, spacecraft commander Charles Conrad, Jr., and pilot Paul J. Weitz spent almost a month on Skylab and safely returned to Earth on 22 June. It was on Skylab that Kerwin had a chance to participate in biomedical experimentation.
IEEE Pulse: Drawing on your background as both an astronaut and a medical doctor, (see “Law in Space”), how has our understanding changed with regard to the effects of space travel on humans?
[accordion title=”Law in Space”]
When explorers lead the way, can the lawyers be far behind? The question of how to practice medicine in space is as old, if not older, then man’s exploration itself. The International Space Station (ISS) is a permanent residence for multiple nationalities. Although those who are selected as astronauts and cosmonauts are generally in peak physical condition, accidents will happen, and there will be unexpected medical events. In fact, these have already occurred. Apollo 13 almost had a catastrophic ending; not only as a result of the equipment disaster, but also as a result of one of the crew members developing sepsis toward the end of the flight. Dental emergencies have also arisen. In fact, Dr. Joe Kerwin performed an early documented medical intervention in space when he performed a full dental evaluation on his crewmember, Commander Pete Conrad, in Skylab in 1973.
The prevention of medical illness in space has occupied the thought of all the international space agencies. In fact, there has been reasoned debate about the advisability of performing prophylactic surgery to remove unnecessary organs that might provide complications in deep space travel far from home. The argument here is that with minimally invasive surgery ubiquitous, prophylactic surgery under controlled conditions is substantially less risky than the situation where diseases would have to be managed far from Earth’s orbit. But there is no surgery without risk, and what if a complication ensues when the surgery was medically unnecessary?
Robotic intervention short-circuits the issue of adequacy of astronaut training. But it brings with it even more complications, some of them with legal implications. The international treaty governing the ISS is clear that each country’s module is operated under its own laws. In the United States, for example, the ISS has been designated a National Laboratory since the enactment of the 2005 NASA Authorization Act. This may affect how liability clams may be reviewed because physicians licensed to practice in any state can practice in a federal health care facility. It is therefore possible that as states increase their legal oversight of professional licensure with the use of telemedicine, those laws could affect the ability of a physician to practice robotic surgery on a patient on the ISS.
But what of commercial space flight, and how about medical complications that may ensue in the practice of robotic surgery? Informed consent may not be enough. These are among the fascinating legal questions that arise as humans escape many of the confines of Earth, many of which we have yet to explore.
Arthur Dula is a space lawyer, a patent attorney, the literary executor for major science fiction author Robert A. Heinlein, and chair and founder of the private spaceflight company Excalibur Almaz.
Sheryl Tatar Dacso is a partner with the firm Seyfarth Shaw, LLP, and certified by the Board of Legal Specialization in Health Law.
[/accordion]
Kerwin: At the beginning, going into space with human beings was something totally new. There was a great deal of speculation about the consequences of weightlessness on the human body, including medical journal articles that forecasted all sorts of dreadful things happening, so the challenge was great.

Once the space program began with Mercury, Gemini, and then Apollo, we had some problems to overcome. One was that nobody knew how to telemeter medical data, so NASA engineers and contractors had to work out how to put the electrodes on a human being to measure pulse, heart rate, respiration, and electrocardiogram, and then get that data conditioned in the right voltage so that it could be added to the communications data—the downlink—coming down to Earth from the spacecraft.
They had to do that in a hurry, and they were very successful. Of course, the data we were looking at from those flights were pretty basic stuff. For instance, “His heart rate is increasing, he must be pretty excited.” (Laughs.) But nonetheless, it was medical telemetry, and it was the first step forward in building an intensive care unit (in hospitals here on Earth). It was great to be in at the beginning of that.
IEEE Pulse: How much human monitoring was done before Skylab?
Kerwin: There just wasn’t enough weight allowance or room to carry experimental equipment, and there wasn’t enough time to train the crew. In addition, the crew wasn’t too enthusiastic about having their bodies monitored during the flight. As a result, much of the data came from exams that the doctors did on the crews before and after the flights. For all of the real questions about the physiology of weightlessness, we had to wait until we had a space station.
IEEE Pulse: How did Skylab change things?
Kerwin: Skylab was a very big, voluminous spacecraft with lots of weight, lots of room, an excellent suite of experiments, but still not much in terms of telemetry. On the other hand, we didn’t really need it because we could take the data in flight, store it, and bring it home with us.
IEEE Pulse: What were some of the biomedical data you collected on board Skylab?
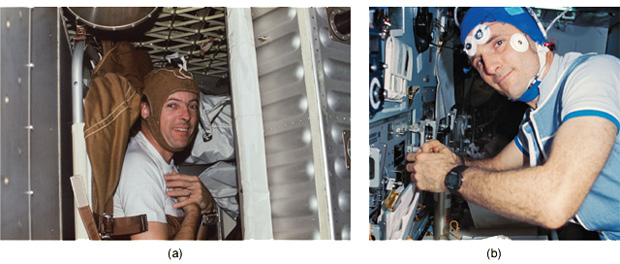
Kerwin: We had vector cardiography, which was a 12-lead electrocardiogram—no big deal, but it had good electrodes and allowed a good collection of data. We also performed sleep electroencephalography, which showed that we slept about an hour less than on Earth but had all four phases; we collected blood once a week for postflight determinations of hormone levels, especially stress-related ones, and red-cell mass; and I tested out “doctor’s office” equipment, including blood counts and differentials. We also measured muscle strength and endurance (arms and legs) pre- and postflight. For exercise-response data, we had a bicycle ergometer and a spirometer to measure inspired and expired gas volumes, but the real development here was an in-flight mass spectrometer to take the gas from the spirometer and measure very accurately the oxygen, nitrogen, and carbon dioxide concentrations. That allowed us to develop metabolic rate as a function of time and workload to compare in-flight data to baseline. This was very important, because guys were coming back from space flight weak, having lost a pint or two of blood and 6–8 lb of weight, with most of it in the lower extremities.
Then, we had an experiment that sounds pretty harmless: mineral balance. What it was, however, was a complete intake and output quantitative measurement experiment never before done outside of a hospital ward. The purpose was to collect information to figure out why astronauts were losing muscle and bone mass while they were in space. For this experiment, we were required to characterize everything that we ate; mass measure everything that was in the package but didn’t get eaten or drunk; collect our urine for 24 hours and then take a carefully shaken-up sample and freeze it, and bring the samples back at the end of the flight; and for feces, freeze-dry it and bring it all back. Using these nutritional parameters, they could then tell if the loss in muscle and bone mass was related to a drop in calcium or protein.
The mineral-balance experiment was actually a son of a gun to design. It was extremely frustrating for the contractors to come up with a system that permitted a high degree of accuracy but was still operable by the crew in less than two or three hours a day, but they ended up doing a good job of biomedical engineering to pull that off.
IEEE Pulse: What challenges were involved in that?
Kerwin: For one thing, they had to come up with the weightless equivalent of bathroom scales that could measure small masses, such as the unused food, as well as large masses. This was done by Dr. Bill Thornton, a very creative engineer who was also a flight surgeon and eventually became an astronaut. What he did was design a device with a strip of stainless steel that was firmly attached to a base and free at the other end. If you flipped the free end, the steel strip would vibrate. The frequency of that vibration was dependent on the mass at the tip of that strip of steel: the heavier the weight, the slower the frequency. That was pretty cool.
IEEE Pulse: Were there any surprises that came out of the experiments?
Kerwin: We were surprised by the human vestibular experiment, which measured your motion-sickness threshold and how that changed in weightlessness. The attempt was to measure your threshold without actually crossing it and barfing. So imagine yourself sitting in your chair and having it rotate at about 10 r/min, and, at the same time, to the beat of a metronome, you have to move your head forward and up, to the right and up, back and up, left and up, and keep on doing that until you have done 150 head movements or until you get sick. We tried that out preflight once and went to the researcher in Pensacola and objected strenuously. He told us we could back off once we got to malaise 2A, which was a certain set of symptoms that you got before you actually got sick.
The surprise came when we did the experiment on the space station. We discovered, to my absolute amazement, that we couldn’t get motion sickness to that degree in weightlessness, even after doing the full 150 head movements. And after we were in space for five days, we had completely acclimatized to the environment. So overall, that experiment was a very nifty job of biomedical engineering with an important negative result.
IEEE Pulse: Were most of the experiments done on Skylab health related?
Kerwin: The principal goals of Skylab were to determine how long people can live and function in weightlessness, whether they can do science while they’re up there, and what accommodations are necessary to keep the astronauts healthy. In that third category, we found that although we returned to Earth healthy, we had, in fact, lost a lot of strength in our arms and legs, and the bicycle ergometer was not enough. We needed more and different kinds of exercise in space. That’s still true today. Although astronauts now have much better resistive exercise devices—a zero-gravity equivalent of weightlifting that delivers some really large forces—and they do minimize bone loss, it’s still a concern, especially as we go from six-months’ duration in space to experimentally a year. (A one-year mission on the International Space Station is planned for 2015.)
IEEE Pulse: When did biomedical experimentation begin expanding beyond human health in weightlessness?
Kerwin: It started coming in during the space shuttle era, before we built the International Space Station (ISS). In the late 1980s or early 1990s, NASA had been flying the shuttle for a week to ten days but saw that they could go to 16, possibly even 21 days, without making any major changes to systems. That opened up possibilities of not only looking at human biomedical problems but also examining other organic and inorganic systems. At about that time, the European Space Agency developed and delivered to NASA what they called the Space Lab, which was a module that went into the cargo bay of the shuttle and provided a large increase in volume and also in electrical power and computer resources for performing other experiments. This was the beginning of real opportunities.
IEEE Pulse: Even after a nearly 50-year career, first with NASA and later with NASA contractors, you continue to be passionate about space. What is it about space that has kept you involved?
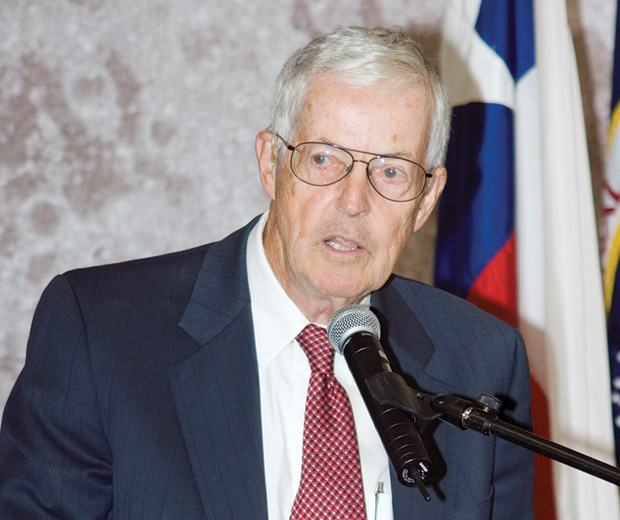
Kerwin: I read science fiction as a kid, and I thought space was a fascinating concept—the idea that you might be able to go off the Earth and into space. That I was actually able to do that as a young adult was mind-blowing. How lucky I was to be alive when I was! So it’s a combination of that and the feeling that this was not only a great adventure for an individual but also that this is something of national importance, that working out the space problems, and getting better at it, and making it routine are good things to do. We still don’t have all the answers, but we do our best. And that’s worth spending your career on.
Jerry Linenger, U.S. Astronaut/Mir Cosmonaut, 1997
In 1997, astronaut Jerry Linenger spent 132 consecutive days orbiting approximately 240 mi above the Earth aboard the Russian space station Mir. During that time, he and the two cosmonauts that made up the crew survived a number of very close calls, including repeated system failures, complete electrical power outages that not only enveloped the crew in total darkness but also left them tumbling out of control through space at some 17,800 mi/h, and a raging fire that spewed blinding and choking smoke and threatened the station itself.
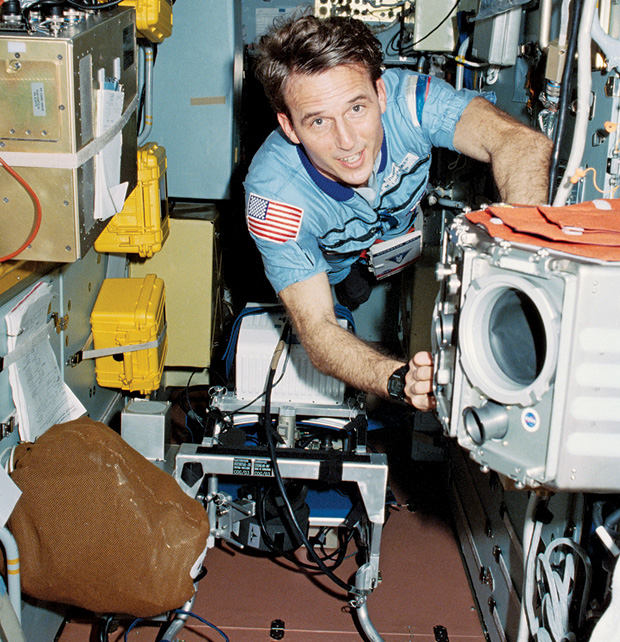
Although he finally returned safely to Earth, Linenger was left with yet another challenge: to recover physically from space travel. This battle, which would continue for many months—much longer than his five-month Mir voyage—was perhaps the most difficult hurdle, and one that could waylay future long-term space travel. He spoke with IEEE Pulse about this obstacle and the additional everyday challenges he encountered while conducting scientific experiments, scrambling to preserve their miniature ecosystem, and trying to maintain his own physical condition aboard a cramped, cluttered, and relentlessly demanding space station.
IEEE Pulse: What was the greatest physical challenge of the weightlessness of space?
Linenger: One of the things I could never convey enough—and you just have to be there to understand it—is that it is effortless in space. If I wanted to go from here to the end of the room, it literally takes this. (Linenger points to the opposite side of the room about 30 ft away, touches a finger to the wall next to him, and barely pushes off.) It’s a fantastic feeling to be able to do flips and 200 somersaults in a row, but you do pay a price, and part of that price is muscle atrophy and bone loss.
That bone loss is something that’s still a possible showstopper when you’re thinking about long missions in space. Most physiological measures dampen out over time, but not bone loss. When you go up into space, for instance, you grow a little bit as your intervertebral spaces and discs fill. You get about an inch taller on the first day, and, by the second, you’re two inches taller, but that’s all. It has limits. Other physiological changes happen, too, but the body adapts and things level out. Bone loss, however, is a straight upward line: you just keep losing bone the whole time you’re up there. Your body basically looks at your bone like an overbuilt I-beam and starts remodeling it, getting rid of excess load, and dumping the calcium from the bone into the blood. Actually, a couple of Russian cosmonauts have passed renal stones because they’re hypercalcemic.
IEEE Pulse: One of your objectives on Mir was to find ways to reduce the amount of bone loss. What did you do?
Linenger: The way that we tried to counter that was with exercise that put stress on the bones. I did two one-hour periods of exercise a day. I mainly used a treadmill. I used a 70-kg plate and a windsurfer harness, and that would yank me down so it felt as if I had a person on my shoulders, and then I’d run at different speeds. Toward the end of my time in space, I added bungee cords (to the treadmill apparatus), and I would also do squats and whatever else I could think of to concentrate on the weight-bearing areas of the hip, lower spine, and the head of the femur.
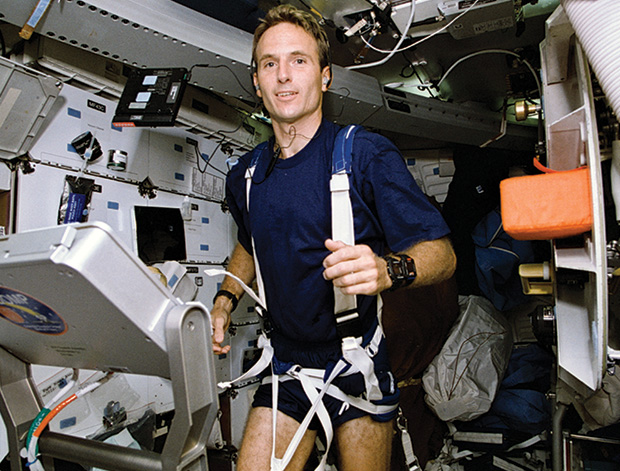
IEEE Pulse: How well did that work?
Linenger: Before I went to space, I tried to be in as good a shape as I could be so that I could use that as a baseline to see what the differential was between preflight and postflight and also what recovery time I needed to get back to 100%. As it turned out, my bone loss from preflight to postflight was 13%. I was very disappointed and almost shocked when I found out I had that much bone loss, because I worked to prevent it.
IEEE Pulse: Is this amount of bone loss typical?
Linenger: It’s hard to say. There’s a lot of individual variation—one astronaut had just 6%, for instance—and there are not that many people who go to space, so there’s really not enough of a sample number to make statistically significant conclusions. That’s one of the reasons astronauts are asked to volunteer for every medical study they can.
One year after my return, I had cut the bone loss down to about 7% in the hips and lower spine, and, at the two-year point, the bone loss was about 2%. Of course, it’s hard to tell what my bone would have lost from normal aging, so I think that 2% loss is acceptable after five months in space.
IEEE Pulse: Since bone loss was a major issue for you during your five months in space, how will that impact extended space travel, such as travel to Mars, where the astronauts will be exposed to weightlessness for an even longer time?
Linenger: Going to Mars will include an approximately six-month transit to get there, a stay for about a year, and then another six months to get back. The vehicle itself will be small, so there will be weight constraints, which means there won’t be room for large treadmills and bicycle ergometers. That does not look good as far as bone loss goes during the transit. The good news is that Mars is (about 39% of) Earth’s gravity, so the astronauts may not lose more once they’re there.
IEEE Pulse: Are there other concerns about travel to Mars?
Linenger: Radiation is a major problem for the human being, with higher cancer risk as the main issue. Personally, my cumulative radiation level is beyond the lifetime accumulation of a nuclear worker, so if my odds of getting cancer were one in a million preflight, they’re now, say, one in 10,000. And that changes depending on your orbit. If you’re up higher, say 340 mi doing a Hubble Space Telescope repair, you’re outside the Van Allen belt, so you have less radiation protection, especially from solar events that emit a lot more radiation hits and heavy nuclei.
The same is true for the electronics. Radiation hits can cause computer bit flips (a 1 becoming a 0, or vice versa), and you can get up to 30 a day just by increasing your altitude and getting outside of the Van Allen belt. If that computer is getting bombarded, eventually you get a bit flip that is significant. As a result, computers have to have self-correcting programs to be able to detect them and to be able to reboot. In fact, there are typically five computers working aboard a space vehicle. If one goes out of whack, the other four eliminate it and then (the ousted computer) tries to reboot and catch back up. And sometimes a radiation hit can actually take the computer down.
IEEE Pulse: Radiation could also, then, be an issue for in-space experiments that have electronic components. From your perspective as an astronaut who has conducted many of those experiments, what other things should the designers of such experiments take into account?
Linenger: It’s a mentally taxing thing to do experiments that you know are valuable, and to do them for scientists around the world who you know are waiting for those results. It’s a lot on your shoulders when you’re up there by yourself with an experiment that you trained on many months earlier, and usually without any feedback about whether you executed it correctly until you get back down to Earth. So if I’m a designer of any of these experiments, I would work in as much redundancy as possible, harden it against radiation if I can, and add self-correcting mechanisms in case the software does get polluted by radiation hits.
IEEE Pulse: With all of the challenges, including some of them being near-fatal catastrophes, do you miss it? Would you go back to space?
Linenger: Obviously, there were close calls when we were up there: a fire, loss of power, failing life-support systems, a collision with an unmanned cargo ship. And then there were just the normal things, like launch, landing, and the dynamic processes where you’ve got to have data and input going in split seconds, and it’s got to be exactly right or you could be dead. You might call that harrowing, but to me, it’s a miraculous thing, and such a privilege to be sitting in a spacecraft that can do what it’s doing. The fact that human beings have figured out the correct way to bring together the electronics, the computer systems, the feedback, the control surfaces, and everything else is just incredible.
About whether I’d go back, I’ve got four kids, but they’re getting older, and one’s going off to college. So, yes, at some point, I would still love to go.
IEEE Pulse: On a Mars mission?
Linenger: When my family’s grown, I think I’d sign up for that one. It was fantastic going to space: a great experience that I have in my back pocket.